 |
|
The
Deccan beyond the plume hypothesis
|
|
|
Summary
The widely accepted mantle
plume model (e.g.,
Morgan, 1981; Richards et al., 1989; Campbell & Griffiths, 1990) postulates that
(i) the currently active Réunion Island, in the
Indian Ocean, is fed by the narrow “tail”
of a mantle plume that rises from the core-mantle boundary,
(ii) the Deccan continental flood basalt (CFB) province
of India originated from the “head” of the
same plume during its early eruptive phase near the
end of the Cretaceous, and (iii) the Lakshadweep-Chagos
Ridge, an important linear volcanic ridge in the Indian
Ocean, is a product of this plume. It is not generally
appreciated, however, that this so-called “classic”
case of a plume contradicts the plume model in many
ways. For example, there is little petrological evidence
as yet that the Deccan source was abnormally hot, and
the short (~ 1.0 – 0.5 Myr) duration claimed by
some for the eruption of the Deccan is in conflict with
recent Ar-Ar age data that suggest that the total duration
was at least ~ 8 Myr (Sheth
et al.,
2001a,b).
The Deccan CFB was associated with the breakup of the
Seychelles microcontinent from India (e.g.,
Mahoney,
1988). Geological and geophysical data from the Deccan
provide no support for the plume model and arguably
undermine it altogether (Sheth, 2005a,b).
The interplay of several intersecting continental rift
zones in India is apparently responsible for the roughly
circular outcrop of the Deccan. The Lakshadweep-Chagos
Ridge, and the islands of Mauritius and Réunion, are located along fracture zones, and the
systematic southerly age progression along the Ridge
(though questioned) may be a result of southward crack
propagation through the oceanic lithosphere. This idea
avoids the problem of a 10° palaeolatitude discrepancy
which the plume model can only solve with the ad hoc
inclusion of mantle roll. Published Ar-Ar age data for
the Lakshadweep-Chagos Ridge basalts have been seriously
questioned (Baksi, 1999, 2005),
and geochemical data suggest that they likely represent
post-shield volcanism (Sheth et al., 2003) and so are unsuitable for hotspot-based plate reconstructions.
“Enriched” isotopic ratios such as higher-than-N-MORB values of 87Sr/86Sr,
observed in basalts of the Ridge and the Mascarene Islands
may mark the involvement of delaminated enriched continental
mantle instead of a plume (Smith, 1993). High values of
the 3He/4He ratio also do not
represent a deep mantle component or plume (Anderson, 1998a;
1998b).
The three Mascarene Islands (Mauritius, Réunion,
and Rodrigues) are not related to the Deccan but reflect
the recent (post-10 Ma) tectonic-magmatic development
of the African Plate.
I relate CFB volcanism
to continental rifting, which often (but not always)
evolves into full-fledged sea-floor spreading (Sheth, 1999a, 2005a).
I ascribe the rifting itself not to mantle plume heads
but to large-scale plate dynamics, possibly aided by
long-term thermal insulation beneath a supercontinent
which may have surface effects similar to those predicted
for “plume incubation” models. Non-plume,
plate tectonic models are capable of explaining the
Deccan in all its greatness. |
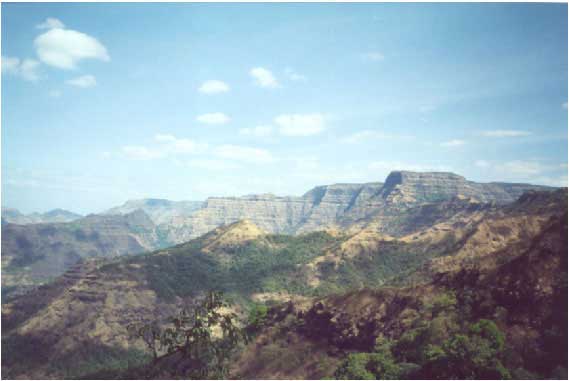
Figure 1. The
1,200-m-thick exposed section through the Deccan
basalt pile at Mahabaleshwar, Sahyadri (Western
Ghats) region. Grand! Photo by Hetu Sheth. |
Since the rapid rise to
dominance of the plume-head/plume-tail model for flood
basalts (Richards et al., 1989; Campbell
& Griffiths, 1990), hundreds of papers have
invoked, or supported, a plume head origin for the Deccan
Traps of India. These papers are in unanimous agreement
on two issues: (i) the Deccan originated from the ancestral
Réunion hotspot
which upwelled beneath India in the late Cretaceous,
and (ii) the hotspot, now located on the African plate,
is fed by a deep mantle plume. The overall appearance
of the Deccan, with its roughly circular outcrop, and
the linear Laccadives-Chagos (more correctly, Lakshadweep-Chagos)
Ridge to the south of India, looks very much like what
is expected for a spherical plume head and a narrow
plume tail (Figures 2 & 3). Nevertheless, the following
observations and deductions suggest that the plume model
is not valid for the Deccan (Sheth, 1999a,b,
2005a).
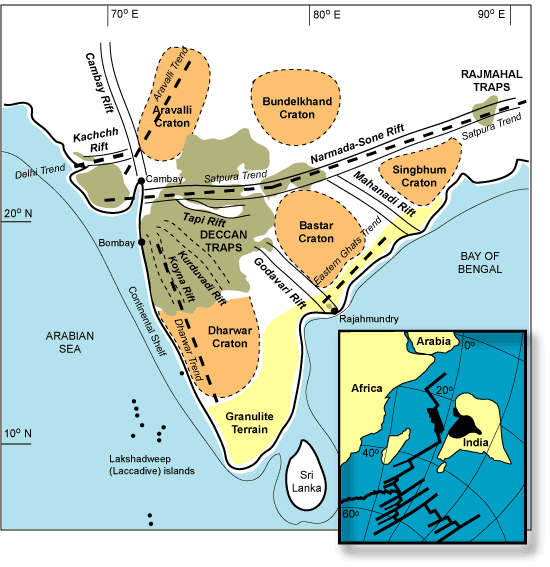
Figure 2. Map showing
the approximate boundaries of the Precambrian cratons
making up the Indian shield (e.g., Pandey & Agrawal,
1999; Naqvi & Rogers, 1987), the granulite terrain,
the Precambrian structural trends (heavy broken lines),
rift zones crossing peninsular India (e.g., Biswas,
1987), and the present outcrop areas of the Deccan and
Rajmahal flood basalts. Inset shows the breakup of the
Seychelles microcontinent, situated along the northern
tip of the Mascarene Plateau (black), from India, soon
after the Deccan flood basalt episode (after Norton
and Sclater, 1979; Mahoney, 1988). The Koyna and Kuruduvadi
“rifts” have been proposed based on gravity
surveys and may represent humps of the granitic basement
rather than rifts.
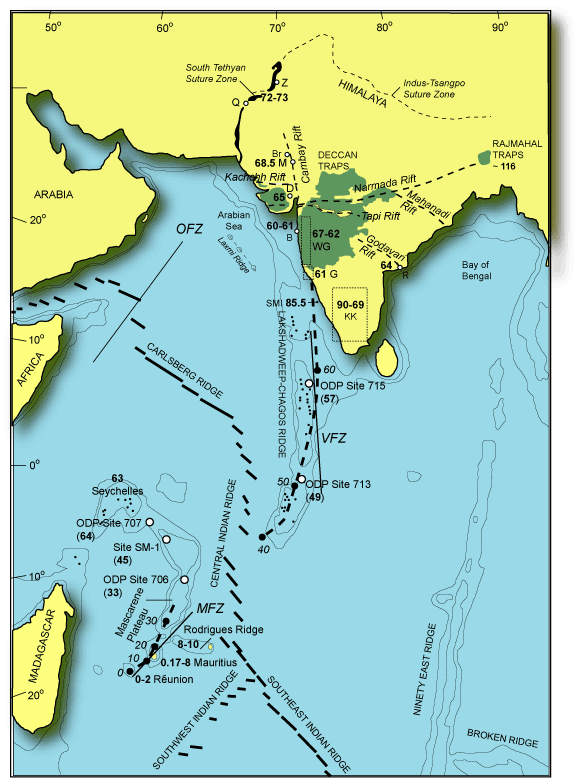
Figure 3. Prominent
structural-tectonic features of southern Asia and the
Indian Ocean basin (based on Mahoney et al., 2002).
Abbreviations for localities are: Q, Quetta; Z, Zhob;
B, Barmer, M, Mundwara; D, Dhandhuka; B, Bombay; R,
Rajahmundry. WG is the Western Ghats region (ages from
Venkatesan et al., 1993 and others). ~ 64 Ma age for
Rajahmundry basalts is from Baksi (2001a). G, ~ 61 Ma
Goa dykes (Widdowson et al., 2000). KK, ~ 90-69 Ma Karnataka-Kerala
dykes (e.g., Radhakrishna et al., 1994; Anil Kumar et
al., 2001). SMI are the St. Mary's Islands volcanics
(85.5 Ma, Pande
et al., 2001), part of the Indo-Madagascar CFB which
in India is otherwise represented by the KK dykes. The
associated flood basalt lavas are not represented or
known in India; there are many Precambrian dyke swarms
throughout southern India as well. 72-73 Ma ages for
Quetta and Zhob rocks and 65 Ma age for Dhandhuka-Botad
lavas are from Mahoney et al. (2002), as also the modelled
hotspot track showing expected ages in Ma. Note the
rift zones underlying the Deccan, and the absence of
any triple junction. OFZ, Owen Fracture Zone; MFZ, Mauritius
Fracture Zone; VFZ, Vishnu Fracture Zone. Click
here for enlargment.
Abnormally hot mantle? There is no evidence for “abnormally hot”
mantle sources for the common and voluminous Deccan
basalts (Figure 4). Some picritic liquids are encountered
in boreholes in the northwestern Deccan and in the Narmada
region (Krishnamurthy et al., 2000). The borehole
lavas were conjectured by Campbell & Griffiths
(1990) to be high-temperature, high-melt-fraction liquids
from the plume axis. Peng & Mahoney (1995),
however, found that they are somewhat alkalic and could
be high-pressure, low-degree melts. The Deccan flood
basalt sequence is best developed in the Western Ghats
region with ~3 km of stratigraphic thickness (Figures
1-3), and picritic basalts are found there, but these
are enriched in cumulus olivine and clinopyroxene and
do not represent liquid compositions. The parental melts
of these picrites are estimated to have contained only
~ 9-10% MgO (Beane & Hooper, 1988; Sheth,
2005b).
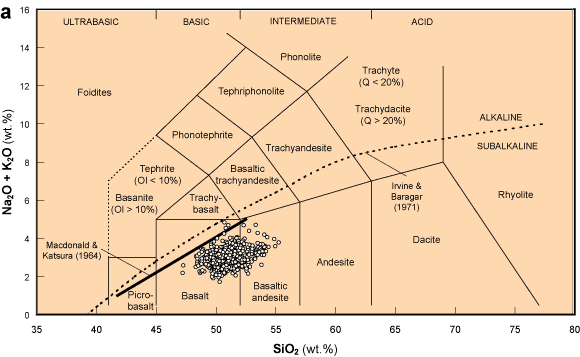
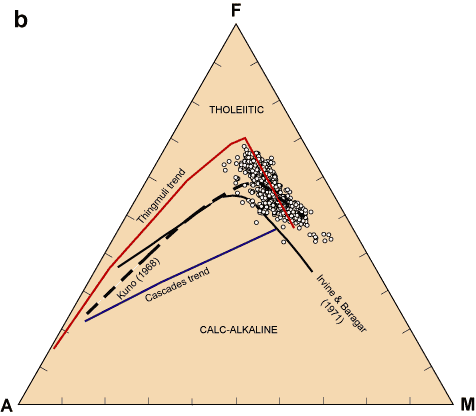
Figure
4. (a) A plot of 624 samples of Deccan basalts of the
Western Ghats (data of Beane, 1988; courtesy J. J. Mahoney)
on the well-known TAS diagram. Note the complete absence
of compositions other than basalt and basaltic andesite,
and the nearly exclusive subalkalic (tholeiitic) nature.
Dividing lines between alkalic and subalkalic fields
proposed by Macdonald & Katsura (1964) and Irvine
& Baragar (1971) are also shown. (b) Plot of the
same samples on the familiar AFM diagram, showing the
Fe enrichment trend typical of tholeiitic basalts. Typical
tholeiite trend (Thingmuli, Iceland) and calc-alkaline
trend (Cascades) are also shown, along with boundaries
between the two fields proposed by Kuno (1968) and Irvine
& Baragar (1971). See Sheth
(2005b) for an extended petrological discussion.
Very short (1 – 0.5 Myr) eruptive duration? Very rapid emplacement
of the Deccan Traps is one of the key arguments for
a plume origin, though also not incompatible with plate-related
and stress-caused mechanisms. The duration of volcanism
has also been one of the most debated issues. Recent
40Ar-39Ar data for trachyte and
basalt flows from Bombay (Sheth et al., 2001a,b)
suggest the total duration to have been no less than
~ 8 – 9 Myr. There may have been a major, rapid,
short-duration eruptive phase in the Western Ghats,
estimated by some to have lasted only 1.0 – 0.5
Myr (e.g., Duncan & Pyle,
1988; Courtillot et al., 1988; Hofmann
et al., 2000), and by others 4 – 5 Myr (Venkatesan
et al., 1993; Pande, 2002). Also, the data
do not always justify the arguments advanced. Allègre
et al. (1999) report an Re-Os isochron age of 65.6
± 0.3 Ma (2σ) for several lava flow samples,
arguing for a very short duration for the volcanism.
That random, non-comagmatic samples collected across
an area 1000 km wide and and at various topographic-stratigraphic
levels should define an isochron is remarkable, but
the goodness-of-fit (F) value for the claimed
isochron, which was not reported, is 22 (Baksi,
2001b); the line is clearly an “errorchron”
(Faure, 1986).
Catastrophic eruption rates? Some authors have explained “the
extremely high lava eruption rates” in CFBs by
hot plume heads, though there is no direct and simple
relationship between melt production and melt eruption
(Th. Thordarson, pers. comm., 2005). A large proportion
of the Deccan basalts comprise pahoehoe compound lava
flows (e.g., Walker, 1970; Bondre et al.,
2004a,b). My own fieldwork at scores of places in the
Deccan, and on the Kilauea volcano, Hawaii (Sheth,
2003), shows that the size and scale of individual
flow units of many large Deccan compound flows are the
same as those of modern Kilauea lava flows (Figure 5).The
large volumes of the individual Deccan lava flows compared
to the Hawaiian flows may reflect in part the great
amount of decompression during India-Seychelles continental
breakup (Figure 2 inset), considerable lengths (40 –
50 km) of the fissure systems (Figure 6; see also Self
et al., 1997), excess source fertility (Sheth,
2005b), mantle volatiles such as CO2
(Presnall
& Gudfinnsson,
2005), and similar features.
|
|
|
Figure 5. (a) The newborn
toe of a compound pahoehoe basalt flow that has emerged
from under the solidified lava crust, as a “breakout”.
The front is about 1 m from the camera and 0.5 m wide.
Ropes are forming in the frontal part and satellite
breakouts emerge at right and left (bright yellow portions).
Kilauea, Hawaii, May 2002. Photo by Hetu Sheth. This
is how I believe the compound pahoehoe flows of the
Deccan were emplaced. (b) Broader view of the actively
inflating pahoehoe compound flow containing the lobe
shown in (a). Note how numerous lobes are juxtaposed
laterally and vertically. I am seen opening with the
hammer the solidified roof of a lobe which yellow-hot
magma (at ~ 1200°C) is filling. Kilauea, May 2002.
Photo by Jyotiranjan Ray. This is how many compound
pahoehoe flows of the Deccan look. Compare with (c).
(c) Section across part of a compound pahoehoe lava
flow of the Deccan, showing the distribution of vesicles
and pipe vesicles. Some 17 flow units are seen. Modified
from Walker (1970). Compare with (a) and (b).
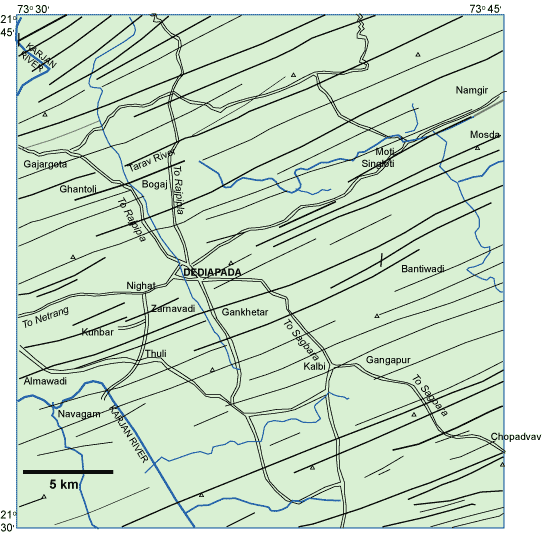
Figure 6. Map of the
Dediapada dyke swarm in the Narmada-Satpura region of
the Deccan (after Krishnamacharlu, 1970). This is one
of the large, spectacular oriented dyke swarms of the
Deccan Traps. Geochemical studies of these dykes are
currently underway. Elevations are in metres.
Internal age progression? None exists within the Deccan (Figure
3). Courtillot & Renne (2003) suggested that
the 60–61 Ma volcanic activity well within the
Deccan (e.g., at Bombay, Sheth et al.,
2001a,b),
was “minor”, and that the duration of Deccan
volcanism was indeed very short. However, (a) this activity
is not minor; large volumes of lava are emplaced in
the subsurface along the west coast, and there is a
scarcity of geochronological data. In comparison, the
Western Ghats section has been heavily sampled and dated.
(b) Whatever its magnitude, the late-persisting volcanism
must be still explained without ad hoc auxiliary
hypotheses. It is not. For example, according to prevalent
views the plume head was all consumed in a quick phase
around 66 – 65 Ma, and the predicted 60 Ma volcanic
basement to the south of India, on top of the Maldives
Ridge, should have formed from the narrow (100 –
200 km wide) plume tail. It is not clear how this plume
tail could produce basalt in Bombay, 1,000 km to the
north, at 60.5 Ma (Sheth et al., 2001b). Suggestions
such as northward dragging of the plume tail by the
plate are ad hoc, and such drag and tilting
would make impossible any systematic age progression
in the first place. Furthermore, if the ~ 69 Ma mafic
dykes reported from Kerala, southernmost India (Radhakrishna
et al., 1994) do represent early Deccan-related
magmatism, as Sheth
(1999b) considered likely, an entirely different,
non-plume, passive, continental-breakup-related model
for Deccan volcanism is even more attractive.
Enriched mantle: plume or continent? Smith (1993) proposed
that ocean-island volcanism is derived from enriched
continental mantle delaminated from a continent rifted
along an ancient suture (see also Lithospheric
delamination page). “Enriched” isotopic
ratios such as higher-than-N-MORB values of 87Sr/86Sr,
for example, are usually taken as plume signatures.
However such compositions may instead mark involvement
of shallow-level, enriched continental mantle. High
values of 3He/4He may also be
explained by shallow models (e.g., Anderson,
1998a, 1998b;
see also Helium fundamentals
page). The ~ 68.5 Ma alkalic complexes (Mundwara,
Barmer) in the northern part of the Deccan province,
related by Basu et al. (1993) to the Réunion
plume based on Sr and He isotopic ratios, could thus
be derived from the continental mantle.
The “enriched” plume model was never
required to explain continental intraplate volcanism,
given the abundance of “enriched” mantle
domains within the continental lithosphere itself. The
plume model was extrapolated to continental magmatism
from the ocean basins based on the world view that the
oceanic mantle was entirely ”depleted“,
MORB-like, convecting and homogeneous. The reasoning
was that anything “enriched” or anomalous
had thus to come from plumes (Anderson, 1996; Smith & Lewis, 1999).
However, if continental mantle is introduced into the
oceanic mantle, e.g., by delamination during continental breakup (e.g., Smith,
1993), enriched plumes are not required to explain either
continental or oceanic intraplate volcanism, and the
whole argument can be turned around. Rather than the
Deccan having formed from a deep mantle plume now located
under Réunion island, Réunion volcanism
may be in part sourced from delaminated Indian continental
mantle.
Mahoney et al. (2002) recently reported Réunion-like elemental
and isotopic compositions for mafic ophiolitic rocks,
dated by them at 72 – 73 Ma, and outcropping in
Pakistan. They opined that some of these may represent
pre-Deccan oceanic seamounts. The associated intrusions
were emplaced in continental shelf-and-slope-type marine
sediments along the northern margin of India. Mahoney
et al. (2002) considered the continental mantle
delamination model, but argued that it does not explain
Réunion-type volcanism occurring on the updrift
side of India at 72 – 73 Ma, and concluded that
the plume model is the most viable option. Notably,
they supported the plume-head-impact model
rather than the plume-head-incubation model, despite
the ~ 8 Myr age gap between the Pakistani rocks and
the 66 – 65 Ma voluminous basalt volcanism of
the Deccan.
Nevertheless, the analyzed intrusions are
located within the boundary of the Indian continental
mantle, and the true oceanic seamounts may not have
been far from the northern margin of India. Continental
mantle delaminated during the early stages of India-Seychelles
breakup could have migrated northward ahead of India
and fed the seamounts built on oceanic lithosphere.
The continent followed behind, and when it converged
upon Asia it simply overrode these seamounts. This is
a better explanation for the observations than the plume
model. If the lateral flow of continental mantle proposed
here seems ad hoc, note that the mechanism of long-distance lateral flow is required
even by the plume model, as for the Rodrigues Ridge
(Morgan, 1981; Figure 3) which is not located along the conjectured Deccan-Réunion
hotspot track and trends roughly E-W. The rocks analyzed
by Mahoney et al. (2002) and Basu et al.
(1993) are undersaturated and alkalic, and have ocean-island-basalt-type
characteristics (e.g., Sr isotopic ratios), but
rather than being melts from a hot plume, they may be
melts of carbonated lherzolite (see Keshav &
Gudfinnsson, 2004).
The “hotspot track”: plume under plate, or crack
propagation? The claimed southerly younging
age progression along the Chagos-Laccadive Ridge and
up to Réunion Island (though duly questioned
by Baksi, 1999, 2005)
does not require a lithospheric plate moving over a
fixed plume. It may be explained by southward crack
propagation through the oceanic lithosphere (see below).
The narrow “hotspot track” may represent
localized melting and magma focusing from a wider area
(the “transform-fault effect”, Langmuir
& Bender, 1984). In support of this, I note
that the Chagos-Laccadive Ridge lies along the Vishnu
Fracture Zone. The Ridge may mark the location of a
major Gondwanic transform (Reeves & de Wit,
2000; Reeves
et al.,
2004).
It is possible that the
current volcanism at Réunion Island may be unrelated
to the Deccan geodynamically, though it taps delaminated
Indian continental mantle brought beneath the African
plate by the ridge jump at ~ 30 Ma (Sheth, 2005a;
see also Burke, 1996). Burke (1996),
a plume proponent, argued that the Deccan plume died
out at 30 Ma and the Réunion plume is a different
plume.
The Cambay triple junction and other fiction. Originally included by
Burke & Dewey (1973) in their world-wide
list of plume-generated triple junctions, the Cambay
triple junction has been popularized by several subsequent
papers supporting the Réunion plume model for
the Deccan. However, the triple junction is not real
(Sheth, 1999b,
2005a;
Figures 2 & 3). Another unfortunate development
is proliferation of model-dependent interpretations
by which every geological and geophysical observation
from the Deccan is interpreted an effect of the Réunion
plume. For example, low-seismic velocity mantle underlying
the Cambay rift of the Deccan is interpreted as a remnant
of the plume (Kennett & Widiyantoro, 1999)
instead of warm, low-density upper mantle welling up
due to rift-related convection. This geophysical feature
may even be a recent (post-Deccan) development (Sheth,
2005a).
Pre-volcanic lithospheric uplift, or lack
thereof? Pre-volcanic lithospheric uplift of up to a few kilometres
is an essential prerequisite for all thermal models
such as the plume model. This is yet another issue on
which specialists of different flood basalt provinces
have come to diametrically opposed conclusions (e.g.,
Czamanske et al.,
1998; He et al., 2003; Tejada
et al., 2004; Saunders et al.,
2005; see also Dhanjori page).
Campbell & Griffiths (1990) cite the Deccan
as a good example of a flood basalt with pre-volcanic
uplift, but the Pachmarhi area mentioned by them as
evidence for this appears instead to show the very opposite
(recent uplift). Pachmarhi is on the Satpura horst between
the Tapi and Narmada rifts. The very youthful landscape
(e.g., kilometre-high escarpments in the basement
Gondwana sandstones) and several planation surfaces
(as high as 1,300 m above MSL) indicate very recent
uplift (Ollier & Pain, 2001).
The
same is true of the Deccan plateau region, where the
Deccan-basement contact is in the subsurface over vast
areas. Major rivers draining the Deccan plateau are
of the antecedent type, i.e., they were in
existence before the Western Ghats (Sahyadri Range)
rose in their way, and the popular dome-flank drainage
picture of the Indian drainage painted by Cox
(1989) is highly speculative. The uplift of the Western
Ghats is post-volcanic and recent (possibly Miocene
and younger), and not pre-volcanic uplift produced by
a plume (Sheth,
2005a). There are two possible interpretations:
(1) Pre-volcanic lithospheric uplift occurred and then
completely decayed and was overprinted by post-volcanic
uplift. This is what plume proponents advocate. (2)
Pre-volcanic uplift never did take place and the plume
explanation is invalid. Option (2) is more plausible,
and there is in fact actual support for it in the form
of an uplifted, extensive planation surface below the
Deccan lavas in central India (Dixey, 1970; see
Sheth,
2005a). Note that the Western Ghats rise much higher
in southern India (the region little or not affected
by Deccan volcanism) than they do in the Deccan plateau
region (Figure 7).
Figure 7. The main
elements of the physiography of the Indian peninsula.
The Western Ghats escarpment is shown by the heavy
broken line. Note the pronounced easterly drainage.
After Ollier & Powar (1985) and Sheth
(2005a).
Palaeolatitudes: true polar wander or crack
propagation? The Deccan lavas erupted at ~ 30°S latitude. Réunion
Island is at 21°S today (Figure 8a). To explain
this significant discrepancy in the framework of the
plume model, some workers have proposed true polar wander
(TPW) of the Earth's mantle (e.g., Vandamme & Courtillot,
1990). In this view, subsequent to the Deccan eruptions,
the Réunion plume remained fixed in the mantle,
while the mantle itself rolled like a ball, inside the
lithospheric shell, in a northerly direction. Such speculation
indicates well the extent of special pleading permitted
within the plume model. Burke (1996) has questioned
this postulated TPW.
I propose a
much simpler alternative to the TPW, illustrated by
the schematic diagram shown in Figure 8b. This is that
the systematically changing palaeolatitudes between
the Deccan and ODP Site 706 (33 Ma) indicate southward
crack propagation in a northward-moving plate with the
condition that the northward plate motion was faster
than the southward crack propagation. The situation
shown in Figure 8b is for a plate in the southern hemisphere.
At time T1, there is an active volcano 1 at the crack
tip at latitude 60°S. Between times T1 and T2, the crack tip has moved
southward by 10°, but because the plate itself has moved north by
20°, the new volcano 2 at the crack tip has the latitude
of 50°S. A similar progression occurs between times T2
and T3. Thus, although the crack tip propagates southward, the palaeolatitudes systematically become more
northerly.
I conclude,
based on diverse evidence, that the Réunion plume
model for the Deccan is wrong.
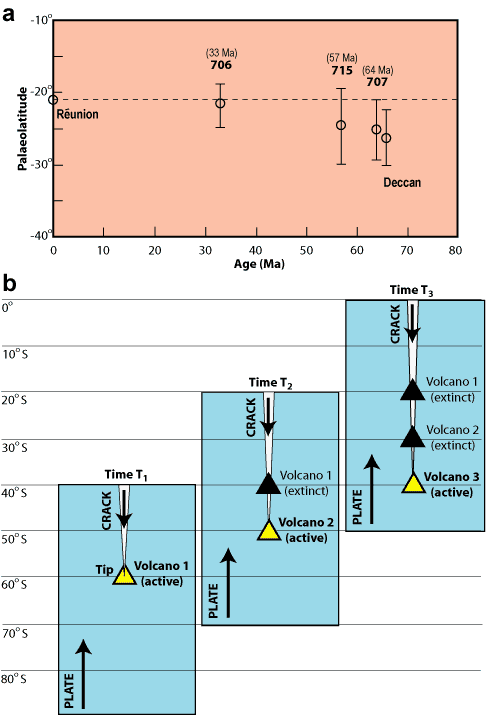
Figure 8: (a)
Palaeolatitude variation from the Deccan to Réunion
Island through the ODP Leg 115 sites (Vandamme &
Courtillot, 1990). (b) Schematic cartoon showing the
development of volcanism resulting from a crack propagating
more slowly southward than the plate moves northward,
in the Southern Hemisphere.
Additional thoughts
Deccan
volcanism was associated with the separation of the
Seychelles microcontinent from India (Figure 2, inset),
and this breakup itself is often ascribed to the Réunion
plume head impact. I propose instead that the breakup
occurred because of prolonged continental extension
and an eventual ridge jump.
One interesting question is whether eclogite, a mantle
rock more fusible than peridotite, could have been a
source in part for the Deccan lavas. The rifted western
continental margin of India follows the NNW-SSE Dharwar
structural trend of the Precambrian southern Indian
shield (e.g., Biswas, 1987). Also, the Narmada
zone that crosses India has been proposed as an ancient
suture between the southern (Dharwar) and northern (Aravalli)
protocontinents (e.g., Naqvi et al., 1974; Naqvi
& Rogers, 1987; Radhakrishna, 1989). Such an ancient
suture may have contained trapped, eclogitized oceanic
crust. Foulger
et al.
(2005) recently proposed such a model for Icelandic
volcanism, and Sheth
(2005b) explored it in considerable detail for the
Deccan. If eclogite constituted a major source for the
Deccan, mantle fertility and not high mantle temperatures
are implicated (see also Yaxley, 2000).
I
have already explained why, despite all problems and
anomalies, the whole Deccan province resembles so much
what a plume-head/plume-plume tail is expected to create.
The interplay of the Deccan rift zones is responsible
for this. Several older sedimentary rift basins underlie
the Deccan (Figures 2 & 3). These are the Cambay,
Kachchh, Narmada-Tapi and Godavari rifts. They are not
arranged radially, are much older than the Deccan, being
of at least Jurassic age, and certainly were not produced
by whatever produced the Deccan. The presence of several
such major rifts means that lithospheric control (Anderson,
1998c), and lithospheric extension (Sheth,
2000) were important in Deccan volcanism. The nearly circular outcrop of the Deccan proper
does not reflect a spherical plume head beneath, but
simply results from the confluence of numerous rift
zones and the continental margin in west-central India.
This is a likely explanation because elsewhere, in the
peripheral/outlier parts of the Deccan along individual
isolated rifts, the lava outcrop is linear or localized
(e.g., the Deccan outliers of the Kachchh and
Rajahmundry areas, the latter being on the Godavari
rift near the east coast of India). The “hotspot
track” on the oceanic crust, as argued above,
may be related to melting and magma focusing along a
southward-propagating fracture. The available seismic
data for Réunion Island support the idea that
its location is related to structural heterogeneity
of the underlying lithosphere (Charvis et al.,
1999; de Voogd & Pontoise, 1999; Hirn, 2002).
In conclusion, a non-plume,
plate-tectonic model involving continental breakup and
related mantle convection and decompression melting,
is suitable for the Deccan. If radial, focused flow
of the upper mantle occurs (instead of vertical flow
as in the plume model), a potentially unlimited volume
of the mantle is available for processing.
The plume model was proposed for the
Deccan more than 30 years ago, when little was known
about the Deccan and about the plume mode of convection.
Today we know a lot more about the Deccan, and the plume
model becomes less tenable as our knowledge grows. The
originators and champions of the plume model had little
or no personal knowledge of the Deccan, and their broad
generalizations should have been put to critical tests
by regional experts on the Deccan. The tendency has
been to assume a plume origin and infer the plume properties
and characteristics based on whatever is required by
the observations, but if volatiles, mantle fertility,
continental geology, and dynamic, evolving plates are
considered, one no longer needs mantle plumes (Sheth,
2005b). The new voices asking for new explanations
(e.g.,
http://www.mantleplumes.org/Deccan2.html)
are a good sign. The plume model for the Deccan has
been around for over thirty years, and has failed. A
new, scientifically tenable non-plume model for the
Deccan could be rapidly developed if only a few of the
many Deccan/flood basalt enthusiasts share the task.
We live in interesting times. |
References
-
Anderson, D. L.,
1996. Enriched asthenosphere and depleted plumes.
Int. Geol. Rev., 38, 1-21.
-
-
-
Anderson, D. L.,
1998c. The EDGES of the mantle. In: The Core-Mantle
Boundary Region. AGU Geodyn. Ser. 28,
255-271.
-
Allègre,
C., Birck, J. L., Capmas, F., Courtillot, V., 1999.
Age of the Deccan Traps using 187Re-187Os
systematics. Earth Planet. Sci. Lett. 170,
199-204.
-
Anil Kumar, Pande,
K., Venkatesan, T. R., Bhaskar Rao, Y. J., 2001.
The Karnataka Late Cretaceous dykes as products
of the Marion hotspot at the India-Madagascar breakup
event: evidence from 40Ar/39Ar
geochronology and geochemistry. Geophys. Res.
Lett., 28, 2715-2718.
-
Baksi, A. K., 1999.
Reevaluation of plate motion models based on hotspot
tracks in the Atlantic and Indian Oceans. J.
Geol., 107, 13-26.
-
Baksi,
A. K., 2005. Radiometric ages of hotspot rocks in
and around the Atlantic, Indian, and Pacific Oceans.
In: Foulger, G. R., Natland, J. H., Presnall, D.
C., Anderson, D. L. (Eds.), Plates, Plumes, and
Paradigms. Geol. Soc. Am. Spec. Pap. 388,
Ch. 5, in press.
-
-
-
Basu, A. R.,
Renne, P. R., DasGupta, D. K., Teichmann, F., Poreda,
R. J., 1993. Early and late alkali igneous pulses
and a high-3He plume origin for the Deccan
flood basalts. Science, 261,
902-906. Beane, J. E., 1988. Flow stratigraphy,
chemical variation and petrogenesis of Deccan flood
basalts from the Western Ghats, India. Ph.D.
dissertation, Washington State Univ., Pullman, U.S.A.
-
Beane, J. E., Hooper,
P. R., 1988. A note on the picrite basalts of the
Western Ghats, Deccan Traps, India. In: Subbarao,
K. V. (Ed.), Deccan Flood Basalts. Mem.
Geol. Soc. Ind. 10, 117-134.
-
Biswas, S. K., 1987.
Regional tectonic framework, structure and evolution
of the western marginal basins of India. Tectonophysics,
135, 307-327.
-
Burke, K. C., 1996.
The African plate. S. Afr. J. Geol., 99,
341-409.
-
Burke, K., Dewey,
J. F., 1973. Plume-generated triple junctions: key
indicators in applying plate tectonics to old rocks.
J. Geol., 81, 406-433.
-
Bondre, N. R., Duraiswami,
R. A., Dole, G., 2004a. Morphology and emplacement
of flows from the Deccan volcanic province, India.
Bull. Volcanol., 66, 29-45.
-
Bondre,
N., Duraiswami, R., Dole, G., 2004b. A brief comparison
of lava flows from the Deccan volcanic province
and the Columbia-Oregon plateau flood basalts: implications
for models of flood basalt emplacement. In: Sheth,
H. C., Pande, K. (Eds.), Magmatism in India through
Time. Proc. Ind. Acad. Sci. (Earth Planet. Sci.),
113, 809-817.
-
Campbell, I. H.,
Griffiths, R. W., 1990. Implications of mantle plume
structure for the evolution of flood basalts. Earth
Planet. Sci. Lett., 99, 79-93.
-
Charvis, P., Laesanpura,
A., Gallart, J., Hirn, A., Lépine, J.-C.,
de Voogd, B., and Pontoise, B., 1999. Spatial distribution
of hotspot material added into the lithosphere under
La Réunion from Wide-angle seismic data.
J. Geophys. Res., 104, 2875-2893.
-
Czamanske, G. K.,
Gurevitch, A. B., Fedorenko, V., Simonov, O., 1998.
Demise of the Siberian plume: palaeogeographic and
palaeotectonic reconstruction from the prevolcanic
and volcanic record, North-central Siberia. Int.
Geol. Rev., 40, 95-115.
-
Courtillot, V. E.,
and Renne, P. R., 2003, On the ages of flood basalt
events. Comp. Rend. Geosci., 335,
113-140.
-
Courtillot, V.,
Féraud, G., Maluski, H., Vandamme, D., Moreau,
M. G., Besse, J., 1988. Deccan flood basalts and
the Cretaceous/Tertiary boundary. Nature,
333, 843-846.
-
Cox, K. G., 1989.
The role of mantle plumes in the development of
continental drainage patterns. Nature, 342,
873-877.
-
De Voogd, B., Pontoise,
B., 1999. Spatial distribution of hotspot material
added into the lithosphere under La RŽunion from
wide-angle seismic data. J. Geophys. Res.,
104, 2875-2893.
-
Dixey, F., 1970,
The geomorphology of Madhya Pradesh, India: West
Commemoration Volume, p. 195-224. (Reprinted in
Subbarao, K. V., 1999. Deccan Volcanic Province.
Geol. Soc. Ind. Mem. 43(2), pp. 575-590.
-
Duncan, R. A., Pyle,
D. G., 1988. Raid eruption of the Deccan Traps at
the Cretaceous/Tertiary boundary. Nature,
333, 841-843.
-
Faure, G., 1986.
Principles of Isotope Geology, 2nd Ed. 589
pp. John Wiley & Sons, New York.
-
-
He, B., Xu, Y.-G.,
Chung, S.-L., Xiao, L., Wang, Y., 2003. Sedimentary
evidence for a rapid, kilometer-scale crustal doming
prior to the eruption of the Emeishan flood basalts.
Earth Planet. Sci. Lett., 213, 391-405.
-
-
Hofmann, C., Féraud,
G., Courtillot, V., 2000. 40Ar/39Ar
dating of mineral separates and whole rocks from
the Western Ghats lava pile: further constraints
on duration and age of the Deccan Traps, Earth
Planet. Sci. Lett., 180, 13-27.
-
Irvine, T. A., Baragar,
W. R. A., 1971. A guide to the chemical classification
of the common rocks. Can. J. Earth Sci.,
8, 523-548.
-
Kennett, B. L. N.,
Widiyantoro, 1999. A low seismic wave-speed anomaly
beneath northwestern India: a seismic signature
of the Deccan plume?. Earth Planet. Sci. Lett.,
165, 145-155.
-
Keshav,
S., Gudfinnsson, G. H., 2004. Silica-poor, mafic
alkaline lavas from ocean islands continents: petrogenetic
constraints from major elements. In: Sheth, H. C.,
Pande, K. (Eds.), Magmatism in India through
Time. Proc. Ind. Acad. Sci. (Earth Planet. Sci.),
113, 723-736.
-
Krishnamacharlu,
T., 1970. Dykes around Dadiapada, Broach district,
Gujarat. In: Aswathanarayana, U. (Ed.), Deccan
Trap and Other Flood Eruptions, Part II. Bull. Volcanol.,
35, 947-956.
-
Krishnamurthy, P.,
Gopalan, K., McDougall, J. D., 2000. Olivine compositions
in picrite basalts and the Deccan volcanic cycle.
J. Petrol., 41, 1057-1069.
-
Kuno, H., 1968.
Differentiation of basaltic magmas. In: Hess, H.
H., Poldervaart, A. (Eds.), Basalts, Vol.
II, pp. 623-688. Wiley Intersci., New York.
-
Langmuir, C. H.,
Bender, J. F., 1984. The geochemistry of oceanic
basalts in the vicinity of transform faults: observations
and implications. Earth Planet. Sci. Lett.,
69, 107-127.
-
Macdonald, G. A.,
Katsura, T., 1964. Chemical composition of Hawaiian
lavas. J. Petrol., 5, 82-133.
-
Mahoney, J. J.,
1988, Deccan Traps. In: Macdougall, J. D. (Ed.),
Continental Flood Basalts, p. 151-194. Kluwer
Acad. Publ., Dordrecht.
-
Mahoney, J. J.,
Duncan, R. A., Khan, W., Gnos, E., McCormick, G.
R., 2002. Cretaceous volcanic rocks of the South
Tethyan suture zone, Pakistan: implications for
the Réunion hotspot and Deccan Traps. Earth
Planet. Sci. Lett., 203, 295-310.
-
Morgan, W. J., 1981.
Hotspot tracks and the opening of the Atlantic and
Indian Oceans. In: Emiliani, C. (Ed.), The Sea,
Vol. 7, pp. 443-487. John Wiley, New York.
-
Naqvi, S. M., Rogers,
J. J. W., 1987, Precambrian geology of India.
223 p. Oxford Univ. Press, New York.
-
Naqvi, S. M., Rao,
V. D., Narain, H., 1974. The protocontinental growth
of the Indian shield and the antiquity of its rift
valleys. Precamb. Res., 1, 345-398.
-
Norton, I. O., Sclater,
J. G., 1979. A model for the evolution of the Indian
Ocean and the breakup of Gondwanaland. J. Geophys.
Res., 84, 6803-6830.
-
Ollier, C., Pain,
C., 2001. The Origin of Mountains. Routledge.
368 pp.
-
Ollier, C., Powar,
K. B., 1985. The Western Ghats and the morphotectonics
of peninsular India: Zeitschrift fuer Geomorphologie,
Supplement-Bd. 54, 57-69.
-
-
Pande,
K., Sheth, H. C., Bhutani, R., 2001. 40Ar/39Ar
age of the St. Mary's Islands volcanics, southern
India: record of India-Madagascar break-up on the
Indian subcontinent, Earth Planet. Sci. Lett.,
193, 39-46.
-
Pandey, O. P., Agrawal,
P. K., 1999. Lithospheric mantle deformation beneath
the Indian cratons. J. Geol., 107,
683-692.
-
Peng, Z. X., Mahoney,
J. J., 1995. Drill-hole lavas from the northwestern
Deccan Traps, and the evolution of Réunion
hotspot mantle. Earth Planet. Sci. Lett.,
134, 169-185.
-
Presnall,
D. C., Gudfinnsson, G. H., 2005. Carbonate-rich
melts in the oceanic low-velocity zone and deep
mantle. In: Foulger, G. R., Natland, J. H., Presnall,
D. C., Anderson, D. L. (Eds.), Plates, Plumes,
and Paradigms. Geol. Soc. Am. Spec. Pap. 388,
Ch. 13, in press.
-
Radhakrishna, B.
P., 1989. Suspect tectono-stratigraphic terrane
elements in the Indian subcontinent. J. Geol.
Soc. Ind., 34, 1-24.
-
Radhakrishna, T.,
Dallmeyer, R. D., Joseph, M., 1994. Palaeomagnetism
and 36Ar/40Ar vs. 39Ar/40Ar
isotope correlation ages of dyke swarms in central
Kerala, India: tectonic implications. Earth
Planet. Sci. Lett., 121, 213-226.
-
Reeves, C.,
de Wit, M. J., 2000. Making ends meet in Gondwana:
retracing the transforms of the Indian Ocean and
reconnecting continental shear zones. Terra
Nova, 12, 272-280.
-
-
Richards,
M. A., Duncan, R. A., Courtillot, V. E., 1989. Flood
basalts and hotspot tracks: plume heads and
tails. Science,
246, 103-107.
-
Saunders, A. D.,
England, R. W., Reichow, M. K., White, R. V., 2005.
A mantle plume origin for the Siberian Traps: uplift
and extension in the West Siberian Basin, Siberia.
Lithos, 79, 407-424.
-
Self, S., Thordarson,
Th., Keszthelyi, L., 1997. Emplacement of continental
flood basalt lava flows. In: Mahoney, J. J., Coffin,
M. F. (Eds.), Large Igneous Provinces: Continental,
Oceanic, and Planetary Flood Volcanism. AGU
Geophys. Monogr. 100, 381-410.
-
-
-
Sheth, H. C., 2000.
The timing of crustal extension, diking, and the
eruption of the Deccan flood basalts. Int. Geol.
Rev., 42, 1007-1016.
-
-
Sheth,
H. C., 2005a. From Deccan to Réunion: no
trace of a mantle plume. In: Foulger, G. R., Natland,
J. H., Presnall, D. C., Anderson, D. L. (Eds.),
Plates, Plumes, and Paradigms. Geol. Soc. Am.
Spec. Pap. 388, Ch. 29, in press.
-
-
-
Sheth,
H. C., Pande, K., Bhutani, R., 2001b. 40Ar-39Ar
age of a national geological monument: the Gilbert
Hill basalt, Deccan Traps, Bombay. Curr. Sci., 80, 1437-1440.
-
-
Smith, A. D., 1993.
The continental mantle as a source for hotspot volcanism.
Terra Nova, 5, 452-460.
-
Smith, A. D., Lewis,
C., 1999. The planet beyond the plume hypothesis.
Earth Sci. Rev., 42, 135-182.
-
Tejada,
M. L. G., Mahoney, J. J., Castillo, P. R., Ingle,
S. P., Sheth, H. C., and Weis, D., 2004, Pin-pricking
the elephant: evidence on the origin of the Ontong
Java Plateau from Pb-Sr-Hf-Nd isotopic characteristics
of ODP Leg 192 basalts, in Fitton, J. G., Mahoney,
J. J., Wallace, P. J., and Saunders, A. D., eds.,
Origin and evolution of the Ontong Java plateau:
Geol. Soc. Spec. Publ. 229, p. 133-150.
-
Venkatesan, T. R.,
Pande, K., Gopalan, K., 1993. Did Deccan volcanism
pre-date the Cretaceous/Tertiary transition?. Earth
Planet. Sci. Lett., 119,181-189.
-
Widdowson, M., Pringle,
M. S., Fernandez, O. A., 2000. A post-K-T boundary
(early Paleocene) age for Deccan-type feeder dykes,
Goa, India.
J. Petrol., 41, 1177-1194.
-
Yaxley, G. M., 2000.
Experimental study of the phase and melting relations
of homogeneous basalt + peridotite mixtures and
implications for the petrogenesis of flood basalts.
Contrib. Mineral. Petrol., 139,
326-338.
-
Vandamme, D., Courtillot,
V., 1990. Palaeomagnetism of Leg 115 basement rocks
and latitudinal evolution of the Réunion
hotspot. Proc. ODP, Sci. Res., 115,
111-117.
-
Walker, G. P. L.,
1970. Compound and simple lava flows and flood basalts.
In: Aswathanarayana, U. (Ed.), Deccan Trap and
Other Flood Eruptions, Part I. Bull. Volcanol.,
35, 513-808.
|
last
updated 29th August, 2006 |
|
|