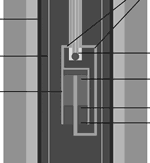 |
Partial
silicate melting in the asthenospheric LVZ: Evidence
from electrical conductivity of hydrous basaltic
melts |
Huaiwei Ni1, Hans Keppler1 & Harald Behrens2
1Bayerisches Geoinstitut, Universität Bayreuth,
95440 Bayreuth, Germany
huaiwei.ni@uni-bayreuth.de, hans.keppler@uni-bayreuth.de
2Institut
für Mineralogie, Leibniz Universität
Hannover, Callinstr. 3, 30167 Hannover,
Germany
h.behrens@mineralogie.uni-hannover.de
This webpage is a summary of: Ni,
Huaiwei, Hans Keppler & Harald Behrens, Electrical
conductivity of hydrous basaltic melts: implications
for partial melting in the upper mantle, Cont.
Min. Pet., 162, 637-650, DOI:
10.1007/s00410-011-0617-4
Introduction
Partial silicate melting
in the asthenospheric LVZ is not a new idea, as the
presence of silicate melt is known to reduce seismic
velocity. Recent magnetotelluric studies (e.g.,
Evans et al., 2005) reported
electrical conductivity as high as 0.1 S/m and strong
conductivity anisotropy in the uppermost asthenosphere.
Available electrical conductivity data of dry basaltic
melt requires at least 5% mantle melting to match
the observed conductivity anomaly, but it would be
difficult for so much silicate melt to stay stable
in the LVZ for a long period of time. Furthermore,
such a degree of melt is not consistent with geochemical
constraints. Some authors (e.g., Wang et al.,
2006) resorted to hydrated mantle minerals, but later
studies (Yoshino et al., 2006) showed that
hydrated olivine cannot account for the observed
anomaly or anisotropy. Carbonate melt is highly conductive
and has also been proposed to be the conductive phase
in the LVZ (Gaillard et al., 2008), but
carbon concentration in the mantle is simply too
low (several tens of ppm) to form enough carbonate
melt, which contains at least 30 wt% CO2. Our study
assesses whether hydrous silicate melt can reconcile
the observations, as the transport properties of
silicate melts are known to be significantly affected
by a few percent dissolved water.
Conductivity measurements
We investigated
the electrical conductivity of both H2O-bearing (0-6
wt% H2O) and CO2-bearing (0.5
wt% CO2) Fe-free basaltic
melts at 2 GPa and 1473-1923 K in a piston cylinder
apparatus (Figure 1). The H2O contents of
starting glasses were analyzed by both Karl Fischer
Titration and FTIR. A glass tube 4 mm long was bracketed
by a Pt rod 0.5 mm in diameter (the inner electrode)
and a Pt95Rh5 tube with an inner
diameter of 3.2 mm (the outer electrode). The two electrodes
were connected to a Solartron 1260 impedance analyzer
using Pt wires 0.35 mm in diameter. Temperature was
monitored using a Pt-Pt90Rh10 thermocouple,
which was not involved in the circuit of conductivity
measurements. The impedance contribution from the Pt
wires was evaluated by a short-circuit experiment.
Samples maintained their shape after experiments but
showed some H2O loss. Conductivities acquired during
the first heating cycle, which were least affected
by H2O loss, are presented here.
Figure 1. Sketch of sample assembly for electrical
conductivity measurements in a piston-cylinder apparatus
(drawn to scale).
The electrical conductivity of basaltic melt depends
on temperature and H2O content (Figure 2)
according to the formula logs = 2.172 – (860.82 – 204.46 w0.5)/(T – 1146.8),
where s is the electrical conductivity in
S/m, T is the temperature in K, and w is
the H2O content in wt%. The effect of small
amounts of CO2 of the order of 0.5 wt% on
electrical conductivity is negligible compared to the
effect of H2O. The positive
effect of water on conductivity is attributed to enhanced
Na mobility and is more pronounced than that of felsic
silicate melts. The conductivity of basaltic melt with > 6
wt% H2O approaches that of carbonatite melt.
Figure 2. Electrical conductivity of volatile-bearing
basaltic melts at 2 GPa.
Implications for partial melting in the LVZ
Our
results can be used to calculate the electrical conductivity
of a partial molten mantle using the upper (HS+) and
lower (HS-) bounds of Hashin & Shtrikman (1962)
or the parallel model. In a depleted mantle source
initially with 125 ppm water, assuming a conductivity
of 0.01 S/m for the solid phases and a bulk water
partition coefficient of 0.006 between minerals and
melt, 2% of melt will result in a bulk mantle conductivity
of 0.1 S/m based on the HS+ model (Figure 3). However,
for plausible higher water contents, stronger water
partitioning into the melt or a more efficient structure
(the parallel model), even less than 1% of hydrous
melt is sufficient to produce the observed conductivity.
Taking this into consideration, our data suggest
that between 0.3 and 2% of hydrous basaltic melt
can account for the observed electrical conductivity
in the LVZ. The melt should be segregated into tube-like
structures, and elongated in the direction of plate
spreading, which would be consistent with both the
observed electric and seismic anisotropy.
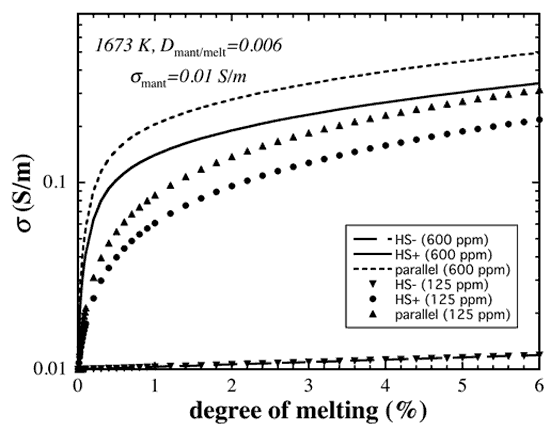
Figure 3. Evolution of the electrical conductivity
of the mantle (with 600 ppm or 125 ppm H2O)
with increasing degree of partial melting, based on
the lower bound (HS-) and upper bound (HS+) of Hashin & Shtrikman
(1962), and the parallel model.
References
-
Evans R.L., Hirth G., Baba K., Forsyth D., Chave A.,
Mackie R., (2005) Geophysical evidence from the MELT
area for compositional controls on oceanic plates. Nature, 437,
249-252.
-
Gaillard F., (2004) Laboratory measurements
of electrical conductivity of hydrous and dry silicic
melts under pressure. Earth Planet. Sci. Lett., 218,
215-228.
-
Hashin Z., Shtrikman S., (1962) A variational
approach to the theory of the effective magnetic
permeability of multiphase materials. J. Appl. Phys., 33,
3125-3131.
-
-
Wang D., Mookherjee M., Xu Y., Karato S.-I., (2006)
The effect of water on the electrical conductivity
of olivine. Nature, 443,
977-980.
-
Yoshino T., Matsuzaki T., Yamashita S., Katsura
T., (2006) Hydrous olivine unable to account for
conductivity anomaly at the top of the asthenosphere. Nature, 443,
973-976.
last updated 23rd
September, 2011 |