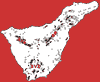 |
Distribution
of basaltic volcanism on Tenerife, Canary Islands:
Origin and dynamics of the rift systems |
Adelina Geyer1 & Joan
Martí2
1Department
of Earth Sciences, University of Bristol, Wills Memorial
Building, Queen’s
Road, BS8 1RJ, Bristol (UK) A.GeyerTraver@bristol.ac.uk
Now at : CIMNE International Center for Numerical Models
in Engineering, Spain ageyer@cimne.upc.edu
2Laboratory
of Simulation of Geological Processes (SIMGEO), Department
of Geophysics and Geohazards, Institute of Earth
Sciences "Jaume Almera",
CSIC, Lluis Solé Sabaris s/n, 08028 Barcelona
(Spain). joan.marti@ija.csic.es
This webpage is a summary of: Geyer,
A., J. Martí, The distribution of basaltic
volcanism on Tenerife, Canary Islands: Implications
on the origin and dynamics of the rift systems, Tectonophysics, 483,
310-326, 2010.
Introduction
In Tenerife, Canary Islands (Figure
1), two rift zones run NW-SE and ENE-WSW and are marked
by parallel rows of aligned cones and eruptive fissures
(e.g., Ancochea
et al., 1990; Carracedo, 1994; Carracedo,
1996; Martí et al., 1996) and fault/dyke
swarms (e.g., Walter & Schmincke,
2002; Walter et al., 2005). These are the
Santiago del Teide (STR) and the Dorsal rifts (DR),
respectively (Figure 1). In the southern part of the
island, the Southern Volcanic Zone (SVZ), basaltic
volcanism is characterized by scattered vents and non-coherently
orientated eruptive fissures. Some authors have associated
the SVZ with a third rift zone orientated N-S, which
may comprise, with the Dorsal and the Santiago del
Teide rifts, a three-armed or “Mercedes Star” rift
structure (Carracedo, 1994; Carracedo, 1996; Walter & Troll,
2003).
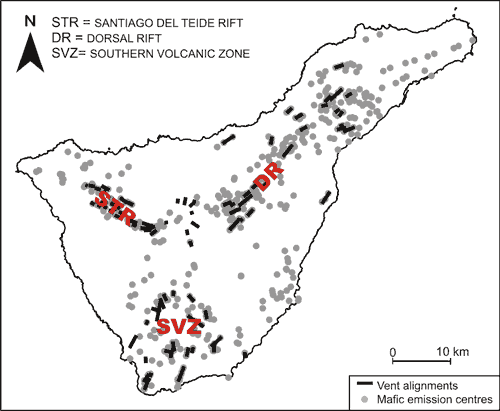
Figure 1: Map of mafic emission
centres and vent alignments on Tenerife Island.
Coordinates refer to 20-km squares of the Spanish
national grid (UTM).
Contrasting genetic explanations have
been proposed for the origin and evolution
of this three-armed rift system. The rift zones are
believed to result either from inflation of the volcano
due to mantle upwelling (Carracedo, 1994,
1996; Figure 2a), or to volcano spreading (Walter,
2003; Figure 2b). The most controversial point is whether
the rift zones have been present throughout the entire
history of Tenerife as a response to mantle upwelling
(Carracedo, 1994, 1996) or, in contrast, whether
they developed later as a result of volcano spreading
(Walter, 2003). In the latter case, the rift
structures would not be directly related to the origin
of the island and their effect on the evolution of
Tenerife would be restricted to more evolved stages.
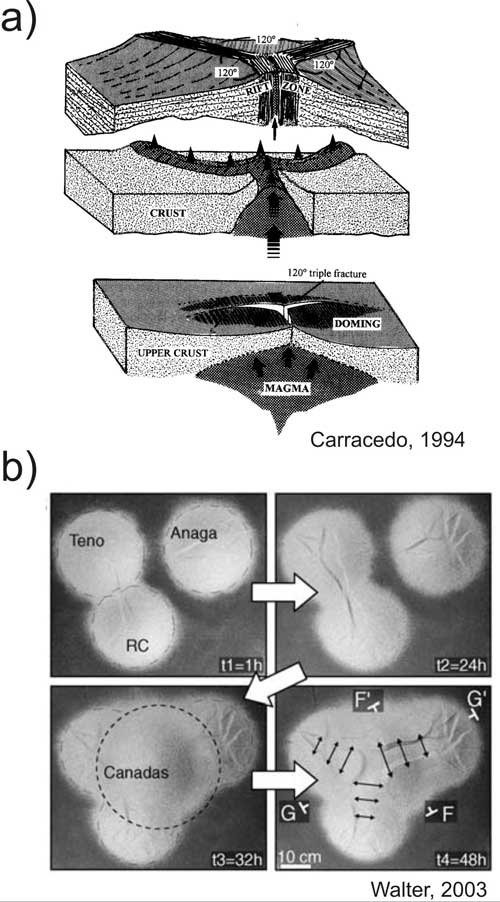
Figure 2: a) A hotspot-based
schematic model for the genesis of a complex three-armed
rift zone on one of the Canary Islands. The concentration
of recent eruptive activity and depressions that may
have been generated by gravitational slides are indicated.
(Modified from Carracedo, 1994). b) Experimental
results of Walter (2003). Three separate sand
cones spread for 32 hours (equivalent to 3 Ma). A rift
is visible between ‘‘Teno’’ and ‘‘Roque
del Conde’’ (RC). Then a fourth cone formed
in the center, deforming the composite edifice for
16 hours (~1 Ma). Three main extensional zones formed.
The best-developed rift is directed to the most distant
cone (here: Anaga).
Geological and tectonic framework of Tenerife
Island
Tenerife is the largest (2058 km2) and highest (3718
m) island of the Canarian archipelago. Ancochea
et al. (1990) proposed that the three old massifs
located at the three corners of the island, Teno in
the northwest (6.7– 4.5 Ma), Anaga in the northeast
(6.5–3.5 Ma), and Roque del Conde in the south
(12–6.4 Ma), represent three independent edifices,
each with its own volcanic history.
Around 3.5 Ma volcanic activity apparently migrated
to the midpoint of the three old edifices forming the
Cañadas edifice, which progressively connected
the subaerial portions of the once-separated edifices.
However, other authors do not share this view (Martí et
al., 1996; Ablay & Kearey, 2000, Araña
et al., 2000; Gottsmann et al., 2008).
The evolution of subaerial volcanism on Tenerife has
been controlled mainly by the ENE-WSW and NW-SE oriented
tectonic trends (Martí et al., 1996;
Figure 1). Evidence comes from geophysical studies
of the oceanic basement around and below the Canary
Islands (Dash & Bosshard, 1969; Bosshard & Macfarlane,
1970; Mezcua et al., 1992; Verhoef et
al., 1991; Roest et al., 1992.; Watts
et al., 1997; Mantovani et al., 2007)
and from dyke distributions and the alignments of recent
mafic vents.
In the SVZ, basaltic volcanism corresponds
to scattered vents that cover an area of over 600 km2 with
a dispersal angle of around 125° relative to Pico
Teide located at the central part of the island (Figure
1). Some of these vents are aligned but the orientation
of the different alignments is highly variable (Figure
1). This area is considered by different authors (e.g.,
Carracedo, 1994; Walter, 2003) to be
a third N-S trending rift zone that corresponds to
the third branch of a three-armed rift structure.
Numerical models
In order to determine
whether the basaltic volcanism at the SVZ corresponds
to the third N-S running branch of a three-armed rift
system or, in contrast, if it just results from the
interaction between the NW-SE and the ENE-WSW rift
zones, we performed 2-D modelling considering the two
main rift zones as two intersecting finite fractures
of 120 m width (Figure 3).
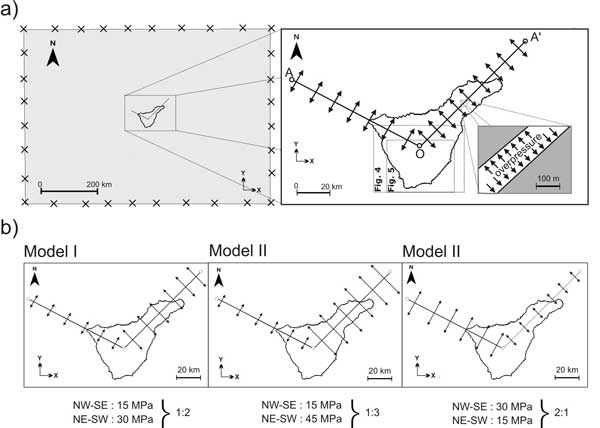
Figure 3: Sketch of the numerical models
calculated. a) Sketch of the whole computational domain
and a zoom in on the area studied, which covers the
whole of Tenerife island. The dashed-line rectangles
mark the areas shown in Figures 4 and 5. A zoom in
on one of the rift axes shows the disposition of the
overpressure applied to the rift limits. The computational
domain is shaded grey. The overpressure assigned to
the boundaries of the model is a requisite for finite-element
calculation. b) Sketch of the overpressure conditions
for the different numerical models.
We assume that the crust behaves as
a linear homogeneous elastic material and we apply
plain strain, i.e.,
the studied area is a section of a very long prismatic
body with standard values of Young’s modulus
E = 40 GPa and Poisson’s coefficient n= 0.25.
The opening of the rift structures was simulated by
excess pressure on the rift axis limits (Figure 3b).
Figure 4 shows the values of σ3 (maximum
tensile stress or minimum compressive stress) and Figure
5 the stress trajectories of σ1 (maximum compressive
stress). The contour fill values of σ3 give us the
area with higher extension and consequently the zone
where emission centres have the highest probability
of appearing. Furthermore, we studied the trajectories
of σ1 which allowed us to infer the
preferred orientation of eruptive fissures and vent
alignments.
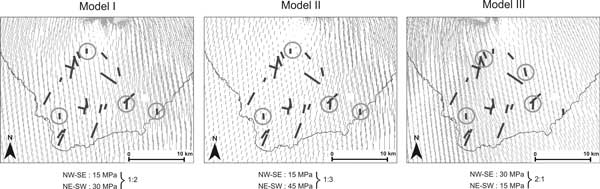
Figure 4: Values of σ3 for
the different numerical examples. Black dots correspond
to mafic emission centres observed in the field.
The figure area is defined in Figures 1 and 2. Click here or on Figure for enlargement.
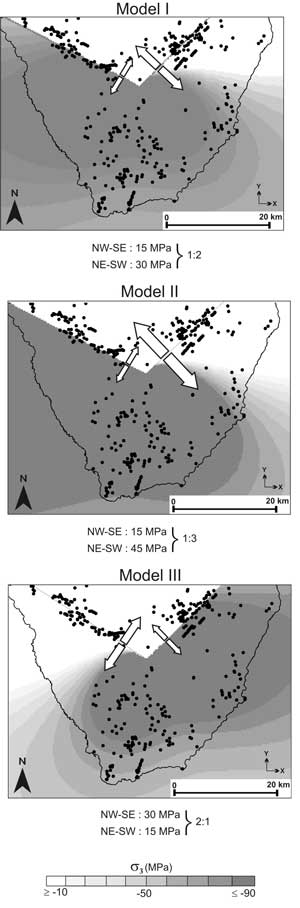
Figure 5: Trajectories of σ1 for the
different numerical examples. Black lines correspond
to eruptive fissures observed in the field. Grey circles
mark those fissures whose orientation is in agreement
with the trajectories of σ1 obtained with the corresponding
numerical model.
In short, the overpressure value modifies the extensive
character of the zone but it is the overpressure relation
between both axes that exerts the main control on the
distribution and location of the extensive area propitious
for the location of basaltic vents in the south of
Tenerife. Furthermore, variations in the pressure ratio
of the rift axis lead to a change in the orientation
of the σ1 trajectories.
Discussion
The third N-S rift zone
and the origin of the Tenerife rift zones
Authors favouring the idea of a three-armed
rift system in Tenerife (Carracedo, 1994,
1996; Walter,
2003) have ignored the fact that the vent alignments
in the south show a relatively wide range of orientations
(Figure 1), implying that not all of them formed under
the same stress field, as shown in the numerical models.
Our numerical results favour the Santiago del Teide
and Dorsal rift zones being related to crustal NW-SE
and ENE-WSW tectonic structures that were present in
the basement on which Tenerife has grown. This agrees
with the results from detailed structural studies on
Tenerife and other Canary islands (e.g., Staudigel
et al., 1986; Marinoni & Pasquarè,
1994; Marinoni & Gudmundsson, 2000; Gee
et al., 2001; Blanco-Montenegro et al.,
2003; Klügel et al., 2005; Muñoz
et al., 2005; Ancochea et al., 2008).
These rifts zones have been used to drive mantle-derived
mafic magmas to the surface during the entire history
of the island and have been active simultaneously or
alternately during different time periods. Thus, they
have played a fundamental role in the origin and evolution
of the island, not only in controlling recent basaltic
volcanism.
Evolution of Tenerife Island
The origin of Tenerife is closely
related to the presence of pre-exiting tectonic structures
in the crust. Volcanic activity began as fissural volcanism
along these structures and the accumulation of the
basaltic series gave rise to the construction of the
composite shield volcano (the Old Basaltic Complex;
Figure 6). We assume that volcanism concentrated along
the NW-SE and ENE-WSW fissures, especially at their
junction, where more eruptives accumulated and thu
differential growth occurred compared to other parts
of the shield. This resulted in the formation of the
dense core of the shield volcano (Boca de Tauce edifice; e.g.,
Ablay & Kearey,
2000; Gottsmann et al., 2008). The increase
in height of the central part of the shield complex
allowed ascending mafic magma to accumulate in the
basaltic construct, forming shallow magma chambers,
evolving phonolitic compositions and driving the construction
of the Tenerife central volcanic complex (the Cañadas
edifice).
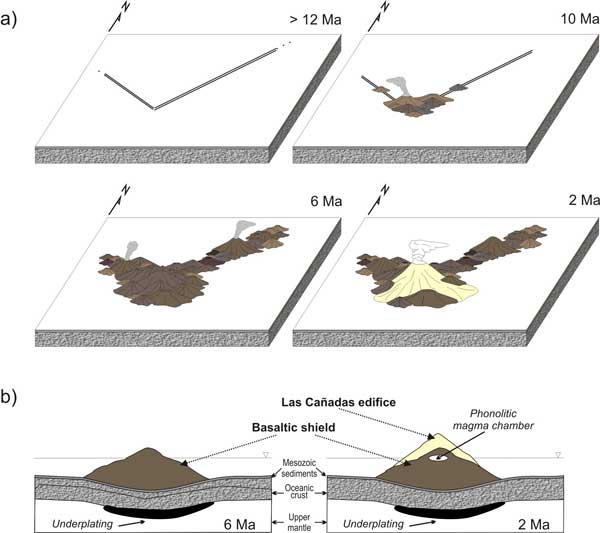
Figure 6: a) Time-spatial evolution
of Tenerife. Dark- and light-coloured edifices correspond
to the Old Basaltic Series and the Cañadas edifice,
respectively. b) Cross-section cutting the Old Basaltic
Series and the Cañadas edifices. The increase
in height of the central part of the shield complex
allowed ascending mafic magmas to stop and accumulate
in the interior of the basaltic construct, forming
shallow magma chambers. There, they evolved phonolitic
compositions and drove the construction of the Tenerife
central volcanic complex (the Cañadas edifice). Click here or
on Figure for enlargement.
The presence of shallow magma chambers during the
construction of the central volcanic complex has not
influenced the dynamics of the rift systems and their
associated stress field. On the contrary, the shallow
magmatic system has been strongly influenced by the
rift structures, as is demonstrated by two facts:
-
the Cañadas phonolitic
dykes, which are clearly associated with the existence
of several shallow magma chambers, show the NE-SW
and ENE-WSW predominant orientations of the two
rift systems (Martí et al., 1994); and
-
these magma chambers formed
successively during the construction of the Cañadas
central complex and fed different volcanic centres
that migrated along the western side of the Dorsal
rift, younging from west to east (Martí & Gudmundsson,
2000).
Relationship between the Canary
Islands and the Atlas Mountains
Regional and local tectonics are relevant
to understanding the evolution of the Canary Island
archipelago as a whole (Hérnandez-Pacheco & Ibarrola,
1973; Anguita & Hernan, 1975; Anguita & Hernan,
2000). In the African continent the Atlas chain (Figure
7), built through the tectonic inversion of a Triassic
and Jurassic intracratonic rift (Jacobshagen et
al., 1988) and associated with the opening of
the North Atlantic, is characterized by post-Cretaceous
Atlas thrusts faults (Mattauer et al., 1977; Proust
et al., 1977; Binot et al., 1986) and
mostly sinistral strike-slip faults (Herbig,
1988; Jacobshagen, 1992). Most faults strike
NNE (in the High Atlas), NE (in the Middle Atlas),
or NW (dispersed though less marked), although there
are also abundant N-S structures. The South Atlas lineament
comprised a discontinuous NNE structure or megashear,
active from Palaeozoic times on. This lineament is
considered to be a part of a newly defined strike-slip
sinistral megastructure more than 1000 km long, the
Trans-Alborán Fault system (TAF; Bousquet & Montenat,
1974; Sanz de Galdeano, 1990) which runs along
the High Atlas and Middle Atlas and crosses the Alborán
(Mediterranean Sea; Figure 7a).
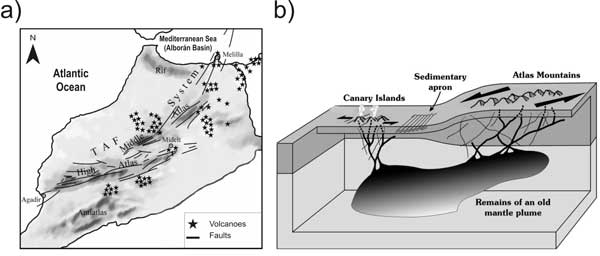
Figure 7: (a) Tectonic alignments
in the Atlas Mountains, (b) schematic model that proposes
a common lineament and melt source for both the Atlas
Mountains and the Canary Islands. Click here or
on Figure for enlargement.
In the Atlantic ocean,
marine geophysicists have found an array of tectonic
structures such as antiforms, synforms and unconformities
(Dillon, 1974; Uchupi
et al., 1976; Dañobeitia & Collette,
1989). A NE striking submarine fault some 50 km long,
with transcurrent (left-lateral) and reverse components
lies between Tenerife and Gran Canaria (Mezcua
et al.,
1992). This fault is similar to the sinistral transcurrent
faults associated with folds on the border of the
Atlas (Piqué et al., 1998).
There
are a number of volcanic areas in the Atlas Mountains
and adjacent zones (Figure 7). Magmatism occurred in
three different periods: Eocene to Oligocene (45-35
Ma), Miocene (14-6 Ma; Middle Atlas) and 1.8-0.5 Ma.
The same rock types as are present in the Atlas Mountains
are also represented in the Canary Islands and the
rock ages are similar as well.
Anguita & Hernán (2000)
suggested a residual fossil
plume under North Africa, the
Canary Islands, and western
and central Europe, on the basis of seismic tomography
(Hoernle & Schmincke,
1993; Figure 7b) [Ed: See also Interpreting
Seismic Velocity page]. Thus, volcanism
is assumed to occur there where an efficient fracture
system allows magma to ascend
(Anguita & Hernán,
2000). Volcanism in Europe, the western Mediterranean,
Iberia, the Canary Islands and Cape Verdes have all
been attributed to this (Hoernle et al.,
1995) [Ed: See also Europe and Italy pages].
Tectonic studies at different islands
have shown trends in fault and volcanic alignments
consistent with oceanic and continental structures e.g.,
Lanzarote, (Marinoni & Pasquarè,
1994) and Gran Canaria, (Anguita et al., 1991).
The main fractures in the islands and ocean floor comprise
two families (Hernandez-Pacheco & Ibarrola,
1973): Atlantic “oceanic” (N160-180°E,
N120-135°E) and African “continental” (strikes:
N20°E, N45°E, N75°E), depending on their
relation with the opening of the Atlantic or the tectonics
of the Atlas range in the African continent (Anguita & Hernán,
1975: Fuster, 1975; Carracedo, 1984; Emery & Uchupi,
1984; Dañobeitia, 1988). The Atlantic
alignment is oriented parallel to transform faults
of the mid-Atlantic ridge (Rona, 1980). Additionally,
alternating periods of magmatism in the islands, and
compression in the Atlas Mountains and Atlantic suggests
that during the tensional periods, the fractures served
as conduits for the ascent of magma (Cousens et
al., 1990; Anderson, 1999; Anguita & Hernán,
2000), while in the compressive epochs they cause uplift
of the islands as sets of flower structures (Figure
3b; Anguita & Hernán, 2000).
Conclusions
Tenerife began with fissural volcanism
along pre-existing crustal structures, progressively
forming a composite shield volcano (the Old Basaltic
Complex). Initial mafic volcanism concentrated
along the NW-SE and ENE-WSW fissures, especially
at their junction. This resulted in formation of
the core of the shield volcano (Boca de Tauce edifice)
and, with time, allowed the ascending mafic magma
to pause and accumulate in the interior of the
basaltic edifice, forming shallow magma chambers.
There, it evolved phonolitic compositions and triggered
the construction of the Tenerife central volcanic
complex (the Cañadas edifice).
In terms of volcanic
hazard related to basaltic volcanism, the present structural
configuration of Tenerife suggests that the maximum
probability of new vents forming is along the rift
zones, preferentially the Santiago del Teide rift,
according to the concentration of the most recent and
historical volcanism.
Acknowledgments
This research was partially
funded with MEC grants BTE-2003-08026 and CGL-2006-13830.
AG is grateful for her MEC post-doctoral fellowship
(2007-0400).
References
-
Ablay, G. J., and P. Kearey (2000), Gravity constraints
on the structure and volcanic evolution of Tenerife,
Canary Islands, J. Geophys. Res., 105(B3),
5783-5796.
-
Ancochea, E., J. M. Fuster, E. Ibarrola,
A. Cendrero, J. Coello, F. Hernan, J. M. Cantagrel,
and C. Jamond (1990), Volcanic evolution of the island
of Tenerife (Canary Island) in the light of new K-Ar
data, J.
Volcanol. Geotherm. Res., 44, 231-249.
-
Ancochea,
E., J. L. Brändle, M. J. Huertas.,
F. Hernan, and R. Herrera (2008), Dike-swarms, key
to the reconstruction of major volcanic edifices:
The basic dikes of La Gomera (Canary Islands), J.
Volcanol. Geotherm. Res., 173, 207-216.
-
Anguita, F., and F. Hernan (1975), A propagating
fracture model versus a hot spot origin for the
Canary islands, Earth Planet. Sci.
Lett., 27, 11-19.
-
Anguita,
F., L. Garcia, F. Colombo, A. Gonzalez, and R.
Vieira (1991), Roque Nublo caldera: a new stratocone
caldera in Gran Canaria, Canary Islands., J.
Volcanol. Geotherm. Res., 47,
45-63.
-
Anguita, F., and F. Hernan (2000), The Canary
Islands origin: a unifying model, J. Volcanol.
Geotherm. Res., 103(1-4), 1-26.
-
Blanco-Montenegro,
I., J. M. Torta, A. García,
and V. Araña (2003), Analysis and modelling
of the aeromagnetic anomalies of Gran Canaria (Canary
Islands), Earth Planet. Sci. Lett., 206,
601-616.
-
Binot, F., G. Dresen, J. Stets, and P. Wurster
(1986), Die Tizi-n'Test- Verwerfungszone im Hohen
Atlas (Marokko). Geol. Rundsch., 75,
647-664.
-
Bousquet, J. C., and C. Montenat
(1974), Prësence de décrochements NE-SW plio-quaternaires
dans les Cordillères bétiques orientales
(Espagne). Extension et signification générale., C.R.
Academic Sciences, Paris 278, 2617-2620.
-
Carracedo, J. C. (1994), The Canary Island:
an example of structural control on the growth of
large oceanic-island volcanoes, J. Volcanol.
Geotherm. Res., 60,
225-241.
-
Carracedo, J. C. (1996), Morphological and
structural evolution of the western Canary Islands:
hotspot-induced three-armed rifts or regional tectonic
trends?, J.
Volcanol. Geotherm. Res., 72, 151-162.
-
Dañobeitia, J. J., and B. J. Collette
(1989), Estudio mediante sísmica de
reflexión de un grupo de estructuras
submarinas situadas al norte y al sur del archipiélago
canario, Acta Geológica Hispánica, 24,
147-163.
-
Dillon, W. P. (1974), Structure
and development of the southern Moroccan continental
shelf., Marine Geology, 16(121-143).
-
Gee,
M. J. R., D. G. Masson, A. B. Watts, and N. C.
Mitchell (2001), Offshore continuation of volcanic
rift zones, El Hierro, Canary Islands, J. Volcanol.
Geotherm. Res., 105, 107-119.
-
-
Gottsmann,
J., A. G. Camacho, J. Martí , L.
Wooller, J. Fernández, A. García, and
H. Rymer (2008), Shallow structure beneath the Central
Volcanic Complex of Tenerife from new gravity data:
Implications for its evolution and recent reactivation, Physics
of the Earth and Planetary Interiors, 168(3-4),
212-230.
-
Herbig, H. G. (1988), Synsedimentary
tectonics in the northernMiddle Atlas (Morocco)
during the Late Cretaceous and Tertiary, in The
Atlas System of Morocco, edited by V. Jacobshagen,
pp. 321-337, Springer, Berlin.
-
Hérnandez-Pacheco,
A., and E. Ibarrola (1973), Geochemical variation
trends between the different Canary Islands in
relation to their geological position, Lithos, 6,
389-402.
-
Hoernle, K., Y. S. Zhang, and
D. Graham (1995), Seismic and geochemical evidence
for large-scale mantle upwelling beneath the eastern
Atlantic and western and central Europe., Nature, 374,
34-39.
-
Hoernle, K. A. J., and H.-U.
Schmincke (1993), The role of partial melting in
the 15-Ma geochemical evolution of Gran Canaria:
A blob model for the Canary hotspot, Journal
of Petrology, 34,
599-626.
-
Jacobshagen, V., R. Brede, M.
Hauptmann, W. Heinitz, and R. Zylka (1988), Structure
and post-Palaeozoic evolution of the central High
Atlas., in The
Atlas System of Morocco., edited by V.
-
Jacobshagen,
pp. 245-271, Springer, Berlin.Jacobshagen, V.
H. (1992), Major fracture zones of Morocco: the
South Atlas fault and the TransAlborán
fault systems., Geol. Rundsch., 81,
185-197.
-
Klügel, A., T. H. Hansteen,
and K. Galipp (2005), Magma storage and underplating
beneath Cumbre Vieja volcano, La Palma (Canary
Islands), Earth
and Planetary Science Letters, 236,
211-226.
-
Marinoni, L. B., and G. Pasquarè (1994),
Tectonic evolution of the emergent part of a volcanic
ocean island: Lanzarote, Canary Islands, Tectonophysics, 239,
111-135.
-
Marinoni, L. B., and A. Gudmundsson (2000),
Dykes, faults and paleostresses in the Teno and Anaga
massifs of Tenerife (Canary Islands), J. Volcanol.
Geotherm. Res., 103, 83-103.
-
Martí,
J., G. J. Ablay, and S. Bryan (1996), Comment on "The
Canary Islands : an example of structural control
on the growth of large oceanic-island volcanoes" by
J.C. Carracedo, J. Volcanol.
Geotherm. Res., 72, 143-149.
-
Martí,
J., and A. Gudmundsson (2000), The Las Cañadas
caldera (Tenerife, Canary islands): an overlapping
collapse caldera generatde by magma-chamber migration, J.
Volcanol. Geotherm. Res., 103 (Special
issue, The geology and geophysics of Tenerife), 161-174.
-
Mattauer,
M., P. Tapponnier, and F. Proust (1977), Sur les
mécanismes de formation des chaînes
intracontinentales. L'exemple des chaînes
atlasiques du Maroc, Bull. Soc. Géol.
Fr., 19, 521-526.
-
Mezcua, J., E.
Buforn, A. Udías, and J. Rueda (1992),
Seismotectonics of the Canary Islands, Tectonophysics, 208,
447-452.
-
Muñoz,
M., J. Sagredo, C. de Ignacio, J. Fernandez-Suarez,
and T. Jeffries (2005), New data (U–Pb, K–Ar)
on the geochronology of the alkaline-carbonatitic
association of Fuerteventura, Canary Islands, Spain, Lithos, 85,
140-153.
-
Piqué, A., P. Le Roy,
and M. Amrhar (1998), ranstensive synsedimentary
tectonics associated with ocean opening: the Essaouira-Agadir
segment of the Moroccan Atlantic margin, Journal
of the Geological Society of London, 155,
913-928.
-
Proust, F., J. P. Petit, and
P. Tapponnier (1977), L'accident du Tizi n'Test
et le rôle
des décrochements dans la tectonique du
Haut Atlas occidental (Maroc). Bull. Soc.
Géol.
Fr., 19, 541-551.
-
Rona, P.A., The central North Atlantic Ocean basin
and continental margins. N.O.A.A. Atlas 3, U.S.D.C,
Washington, DC (1980).
-
Sanz de Galdeano,
C. (1990), La prolongación hacia el sur
de las fosas y desgarres del norte y centro de
Europa: una propuesta de interpretación., Rev.
Soc. Geol. Esp., 3, 231-241.
-
Staudigel, H., G. Feraud, and G. Giannerini
(1986), The story of inttrusive activity on the island
of La Palma (Canary Islands), J. Volcanol. Geotherm.
Res., 27, 229-322.
-
Uchupi, E., K.
O. Emery, C. O. Bowin, and J. E. Phillips (1976),
Continental margin off western Africa: Senegal
to Portugal, Am. Assoc. Pet. Bull., 60,
809-878.
-
Walter, T. R.,
and H.-U. Schmincke (2002), Rifting, recurrent
landsliding and Miocene structural reorganization
on NW-Tenerife (Canary Islands), International
Journal of Earth Science, 91, 615-628.
-
Walter,
T. R., and V. R. Troll (2003), Experiments on rift
zone evolution in unstable volcanic edifices, J.
Volcanol. Geotherm. Res., 127, 107-120.
-
Walter,
T. R., V. R. Troll, B. Cailleau, A. Belousov, H.
U. Schmincke, F. Amelung, and P. Van den Bogaard
(2005), Rift zone reorganization through flank instability
in ocean island volcanoes: an example from Tenerife,
Canary Islands, Bulletin of Volcanology, 67,
281-291.
last updated 19th
March, 2010 |