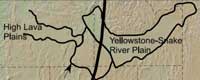 |
Missing Pb, High 3He/4He, Ancient Sulfides and Continent Formation |
Shichun Huang1, Cin-Ty A. Lee2 & Qing-Zhu Yin3
1Department of Geoscience, University of Nevada, Las Vegas, shichun.huang@unlv.edu
2Department of Earth Science, Rice University, ctlee@rice.edu
3Department of Earth and Planetary Sciences, University of California, Davis, qyin@ucdavis.edu
This webpage is a summary of: Huang, Shichun, Cin-Ty A. Lee & Qing-Zhu Yin, Missing Lead and High 3He/4He in Ancient Sulfides Associated with Continental Crust Formation, Scientific Reports, 4, DOI: 10.1038/srep05314
Introduction
Continent formation is a major process that fractionates the Earth. It generates complementary reservoirs that may be sampled by ocean island basalts (OIBs) and mid-ocean ridge basalts (MORBs) (e.g., Hofmann, 1988; Jacobsen, 1988). For example, Parman (2007) found that the pulses of continent formation, represented by age distribution of continental zircons, are couple with 3He/4He distribution peaks in OIBs and MORBs. Lee et al. (2012) found that continental crust is depleted in Cu, and they attributed this Cu depletion to fractionation of sulfide-rich mafic cumulates under arcs during continent formation. Since Cu is highly compatible in sulfides, sulfide-rich mafic cumulates formed during arc magma evolution are enriched in Cu. These sulfide-rich mafic cumulates are then removed from continental crust because of their higher density, a process called delamination, which in turn, results in a felsic and Cu-poor composition for continental crust. Such sulfide-rich mafic cumulates are delaminated into the mantle or stay at the bottom of the continental lithosphere, and may be sampled by OIBs or continental flood basalts (CFBs) via mantle plumes, small-scale convective instabilities, or melting during lithospheric heating and extension.
What are the distinctive geochemical signatures of such sulfide-rich mafic cumulates?
Sulfides are enriched in Pb and depleted in U and Th (e.g., Hart & Gaetani, 2006). Based on published noble gas solubility studies, which show that the solubility of a noble gas in a liquid is a function of the macroscopic surface tension of the liquid (e.g., Uhlig, 1937; Blander et al., 1959; Jambon et al., 1986; Lux, 1987), Huang et al. (2014) showed that He solubility in sulfide melt is close to that in basaltic melt. Consequently, they argue that the sulfide-rich mafic cumulates are also enriched in He. That is, sulfide-rich mafic cumulates are characterized by low (U+Th)/Pb and (U+Th)/He, and they would “freeze” in the 3He/4He, 206Pb/204Pb and 207Pb*/206Pb* ratios of the ambient mantle at the time of their formation. (207Pb*/206Pb* measures the ratio of radiogenic ingrowth of 207Pb and 206Pb since the formation of the Earth.) If formed early in Earth’s history, they would be characterized by high 3He/4He and 207Pb*/206Pb*, and low 206Pb/204Pb, i.e., unradiogenic He and Pb isotopic signatures.
Are such distinctive geochemical signature observed in OIBs and CFBs?
Jackson et al. (2010) argued that at Baffin Island, high 3He/4He is associated with an ancient Pb isotopic signature, i.e., low 206Pb/204Pb and high 207Pb*/206Pb*. Recently, Huang et al. (2014) showed that 3He/4He is negatively correlated with 206Pb/204Pb and positively correlated with 207Pb*/206Pb* in OIBs (Figure 1).
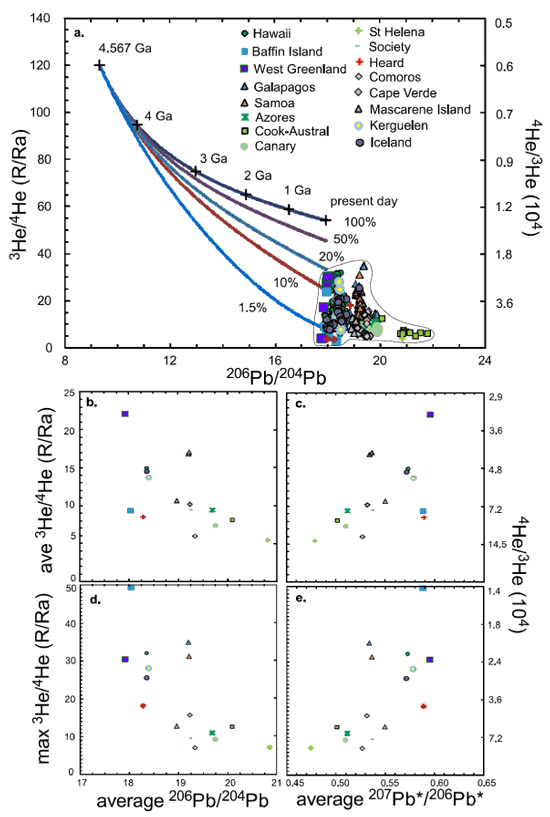
Figure 1: a: 206Pb/204Pb vs. 3He/4He for sulfides formed at different ages and ocean island basalts (OIBs) and continental flood basalts (CFBs). Lines are cross plots of 206Pb/204Pb vs. 3He/4He for sulfides formed at different ages. Since sulfides have very low U/Pb and (U+Th)/He ratios, they preserve the mantle 206Pb/204Pb, 207Pb*/206Pb* and 3He/4He signatures at the time they formed. In OIBs, high-3He/4He is associated with low-206Pb/204Pb. b, c: 206Pb/204Pb and 207Pb*/206Pb* vs. 3He/4He for the average of each OIB-CFB suite. No age-corrections were applied to the 3He/4He of Baffin Island and West Greenland. Consequently the plotted values are their minima. d, e: average 206Pb/204Pb and 207Pb*/206Pb* vs. maximum 3He/4He of each OIB-CFB suite.
Of particular interest, Huang et al. (2014) also observed a similar correlation in Yellowstone-Snake River Plain basalts (Figure 2), one of the few regions of continental intraplate volcanism that has been extensively studied for Pb and He isotopes. This volcanic track has been postulated to be formed by the Yellowstone plume (e.g., Hanan et al., 2008). Along this track, 3He/4He and 207Pb*/206Pb* increase and 206Pb/204Pb decreases from west to east. Lavas erupted on the older Precambrian North American Craton have higher 3He/4He and 207Pb*/206Pb*, and lower 206Pb/204Pb while lavas emplaced through the younger Mesozoic accreted terrains have lower 3He/4He and 207Pb*/206Pb*, and higher 206Pb/204Pb. Such correlations may reflect sampling of sulfide-rich cumulates with different ages, i.e. Precambrian vs. Mesozoic.
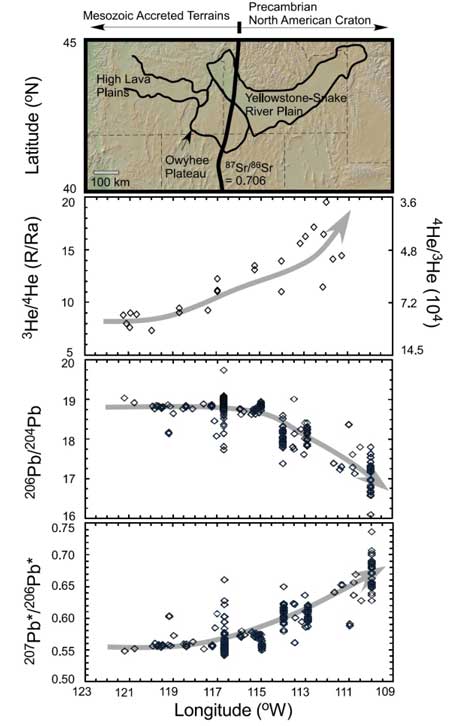
Figure 2: 206Pb/204Pb, 207Pb*/206Pb* and 3He/4He vs. longitude for basalts from the Northwestern United States. High-3He/4He is associated with low-206Pb/204Pb. The 87Sr/86Sr = 0.706 line indicates the boundary of the North American Craton.
The sulfide-rich mafic cumulate model also provides alternative interpretations for two long-lasting questions in the field of mantle geochemistry–the origin of high-3He/4He, and the classic “Pb isotope paradox”.
Many OIBs have 3He/4He higher than that in MORBs, which sample the Earth’s upper mantle. The origin of the high 3He/4He mantle reservoir is the center of many debates. Since 4He is mostly produced by U-Th-Pb decay, a high 3He/4He signature requires an ancient reservoir with low (U+Th)/He. In most cases, He escapes to the atmosphere. Therefore, in the traditional view, any mantle process would increase the (U+Th)/He ratio. Consequently, the high 3He/4He reservoir has been postulated to be the primitive mantle (e.g., Kurz et al., 1983; Jackson et al., 2010), partially degassed, or less processed primitive mantle (e.g., Class & Goldstein, 2005; Porcelli & Elliott, 2008; Gonnermann & Mukhopadhyay, 2009). According to another view, a low (U+Th)/He reservoir may form by dissolving or diffusing He into a depleted mantle reservoir with low U+Th abundances (e.g., Parman et al., 2005; Albarède, 2008; Hart et al., 2008; Jackson et al., 2013). That is, He added to a depleted mantle reservoir forms a low (U+Th)/He reservoir.
Although one of the best studied elements, the Pb budget of the Earth is not fully understood. Major crustal and mantle reservoirs have Pb isotopes more radiogenic than bulk silicate Earth. Consequently, there must be a reservoir, whose location is unknown, with unradiogenic Pb (Allègre, 1968). This is the classic “Pb isotope paradox”. The missing unradiogenic Pb has been argued to be in the lower continental crust (e.g., O’Nions et al., 1979) or dissolved in the core early in Earth’s history (e.g., Allègre et al., 1995; Wood & Halliday, 2010). However, granulite terranes are not sufficiently unradiogenic (e.g., Zartmen & Haines, 1988; Rudnick & Goldstein, 1990; Rudnick & Gao, 2003) and the timing of core formation implied by Pb sequestration into the core is too young compared to that determined by 182Hf-182W systematics (Kleine et al., 2002; Yin et al., 2002).
In the model of Huang et al. (2014), ancient sulfide-rich mafic cumulates are the host of both the “missing Pb” and the high-3He/4He signature. Thus, the high-3He/4He, high-207Pb*/206Pb* and low-206Pb/204Pb reservoirs in the OIB-CFB sources may correspond to the complements of continent formation rather than primordial parcels of mantle that have remained unprocessed through Earth’s entire history.
References
- Albarède, F. Rogue Mantle Helium and Neon. Science 319, 943-945, doi:10.1126/science.1150060 (2008).
- Allègre, C. J. Behavior of Uranium-Thorium-Lead Systems in Upper Mantle and Model of Mantle Development during Geological Times. Earth and Planetary Science Letters 5, 261-269, doi: 10.1016/S0012-821x(68)80050-0 (1968).
- Allègre, C. J., Manhes, G. & Gopel, C. The Age of the Earth. Geochimica et Cosmochimica Acta 59, 1445-1456, doi:10.1016/0016-7037(95)00054-4 (1995).
- Blander, M., Grimes, W. R., Smith, N. V. & Watson, G. M. Solubility of Noble Gases in Molten Fluorides. 2. In the Lif-Naf-Kf Eutectic Mixture. Journal of Physical Chemistry 63, 1164-1167, doi:10.1021/J150577a033 (1959).
- Class, C. & Goldstein, S. L. Evolution of helium isotopes in the Earth's mantle. Nature 436, 1107-1112, doi:10.1038/Nature03930 (2005).
- Gonnermann, H. M. & Mukhopadhyay, S. Non-equilibrium degassing and a primordial source for helium in ocean-island volcanism. Nature 449, 1037-1040, doi:10.1038/Nature06240 (2007).
- Hanan, B. B., Shervais, J. W. & Vetter, S. K. Yellowstone plume-continental lithosphere interaction beneath the Snake River Plain. Geology 36, 51-54, doi:10.1130/G23935a.1 (2008).
- Hart, S. R. & Gaetani, G. A. Mantle Pb paradoxes: the sulfide solution. Contributions to Mineralogy and Petrology 152, 295-308, doi:10.1007/s00410-006-0108-1 (2006).
- Hart, S. R., Kurz, M. D. & Wang, Z. Scale length of mantle heterogeneities: Constraints from helium diffusion. Earth and Planetary Science Letters 269, 508-517, doi:10.1016/j.epsl.2008.03.010 (2008).
- Hofmann, A. W. Chemical differentiation of the Earth: the relationship between mantle, continental crust, and oceanic crust. Earth and Planetary Science Letters 90, 297-314 (1988).
- Huang, S., Lee, C.-T. A. & Yin, Q.-Z. Missing Lead and High 3He/4He in Ancient Sulfides Associated with Continent Crust Formation. Scientific Reports 4, 5314, doi:10.1038/srep05314 (2014).
- Jacobsen, S. B. Isotopic and chemical constraints on mantle-crust evolution. Geochimica et Cosmochimica Acta 52, 1341-1350 (1988).
- Jackson, M. G. et al. Evidence for the survival of the oldest terrestrial mantle reservoir. Nature 466, 853-856, doi:10.1038/Nature09287 (2010).
- Jackson, C. R. M., Parman, S., Kelley, S. P. & Cooper, R. F. Noble gas transport into the mantle facilitated by high solubility in amphibole. Nature Geoscience 6, 562-565, doi:10.1038/Ngeo1851 (2013).
- Jambon, A., Weber, H. & Braun, O. Solubility of He, Ne, Ar, Kr and Xe in a Basalt Melt in the Range 1250-1600-Degrees-C. Geochimica et Cosmochimica Acta 50, 401-408, doi:10.1016/0016-7037(86)90193-6 (1986).
- Kleine, T., Munker, C., Mezger, K. & Palme, H. Rapid accretion and early core formation on asteroids and the terrestrial planets from Hf-W chronometry. Nature 418, 952-955, doi:10.1038/Nature00982 (2002).
- Kurz, M. D., Jenkins, W. J., Hart, S. R. & Clague, D. Helium isotopic variations in volcanic rocks from Loihi Seamount and the Island of Hawaii. Earth and Planetary Science Letters 66, 388-406 (1983).
- Lee, C.-T. A. et al. Copper Systematics in Arc Magmas and Implications for Crust-Mantle Differentiation. Science 336, 64-68, doi:10.1126/science.1217313 (2012).
- Lux, G. The Behavior of Noble-Gases in Silicate Liquids - Solution, Diffusion, Bubbles and Surface Effects, with Applications to Natural Samples. Geochimica et Cosmochimica Acta 51, 1549-1560, doi:10.1016/0016-7037(87)90336-X (1987).
- O'Nions, R. K., Evensen, N. M. & Hamilton, P. J. Geochemical modeling of mantle differentiation and crustal growth. Journal of Geophysical Research: Solid Earth 84, 6091-6101, doi:10.1029/JB084iB11p06091 (1979).
- Parman, S. W., Kurz, M. D., Hart, S. R. & Grove, T. L. Helium solubility in olicine and implications for high 3He/4He in ocean island basalts. Nature 437, 1140-1143, doi:10.1038/nature04215 (2005).
- Parman, S. W. Helium isotopic evidence for episodic mantle melting and crustal growth. Nature 446, 900-903, doi:10.1038/Nature05691 (2007).
- Porcelli, D. & Elliott, T. The evolution of He Isotopes in the convecting mantle and the preservation of high 3He/4He ratios. Earth and Planetary Science Letters 269, 175-185, doi:10.1016/j.epsl.2008.02.002 (2008).
- Rudnick, R. L. & Goldstein, S. L. The Pb isotopic compositions of lower crustal xenoliths and the evolution of lower crustal Pb. Earth and Planetary Science Letters 98, 192-207, doi:10.1016/0012-821X(90)90059-7 (1990).
- Rudnick, R. L. & Gao, S. Composition of the Continental Crust. Treatise on Geochemistry 3. Editor: Roberta L. Rudnick. Executive Editors: Heinrich D. Holland and Karl K. Turekian. ISBN 0-08-043751-6. 1-64, doi:10.1016/b0-08-043751-6/03016-4 (Elsevier 2003).
- Uhlig, H. H. The solubilities of gases and surface tension. Journal of Physical Chemistry 41, 1215-1225, doi:10.1021/J150387a007 (1937).
- Wood, B. J. & Halliday, A. N. The lead isotopic age of the Earth can be explained by core formation alone. Nature 465, 767-U764, doi:10.1038/Nature09072 (2010).
- Yin, Q. Z. et al. A short timescale for terrestrial planet formation from Hf-W chronometry of meteorites. Nature 418, 949-952, doi:10.1038/Nature00995 (2002).
- Zartman, R. E. & Haines, S. M. The Plumbotectonic Model for Pb Isotopic Systematics among Major Terrestrial Reservoirs - a Case for Bi-Directional Transport. Geochimica et Cosmochimica Acta 52, 1327-1339, doi:10.1016/0016-7037(88)90204-9 (1988).
last updated 10th
September, 2014 |