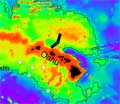 |
Heat
Flow on Hotspot Swells |
Robert N. Harris
College of Oceanic and
Atmospheric Sciences, Oregon State University
rharris@coas.oregonstate.edu
Two assumptions that commonly guide
the interpretation of heat flow data collected on
hotspot swells are the choice of a reference model
and that values of surface heat flow represent lithospheric
heat flow. In an earlier webpage on this
site, Stein
& Stein (2005) discuss assumptions related
to the choice of a reference model. Because
the expected thermal anomalies are small, anomalous
heat flow depends strongly on the reference model. Stein
& Stein (1993) reviewed average heat flow
values over hotspot swells relative to their model
(GDH1 for global depth and heat flow as a function
of crustal age; Stein & Stein,
1992)
and concluded that while hotspot swells are anomalously
shallow, heat flow is not anomalously high. Based
on the presence of a bathymetric anomaly and lack
of a heat flow anomaly, they argued that hot spot
swells are dynamically, not thermally, supported.
The second assumption
is that heat flow values represent conductive heat
transfer through the lithosphere so that these values
can be used to infer the thermal state at the base
of the lithosphere. In fact until very recently
heat flow surveys over hotspot swells were designed
assuming conductive heat transport. As a consequence
heat flow was thought to vary at the long wavelengths
associated with a sublithospheric thermal plume. With
this supposition in mind, and the tradeoff between
covering large areas with limited ship time, early
surveys were designed to consist of about seven or
so ~10 x 10 km areas, each with multiple (10-20) heat
flow determinations. Large distances (of the
order of 100 km) generally separate these areas and
heat flow determinations were averaged together to
cancel variability.
Averages of heat-flow
determinations from these widely spaced areas collected
for the purpose of measuring the thermal flux from
hotspots have been published at four oceanic swells:
Hawaii (Von Herzen et al., 1982, 1989), Bermuda
(Detrick et al., 1986), Crozet (Courtney
& Recq, 1986), and Cape Verde (Courtney
& White, 1986). Harris
& McNutt (2007) analyze inter-site variability
of individual heat flow determinations. They
show that inter-site variability is generally larger
than instrumental noise as indicated by repeat measurements
at the same site, and that as the volcanic edifice
is approached the scatter of values at each site increases. Harris
& McNutt (2007) argue that this pattern is
inconsistent with conductive heat transfer and that
the most likely source of perturbation is advective
fluid flow.
Discriminating
between environments where heat is transferred
conductively or advectively requires closely
spaced heat flow determinations (1-2 km) collocated
with seismic reflection profiles. Only
Hawaii (Harris et al.,
2000a) and Reunion
(Bonneville et al.,
1997) have surveys
meeting these requirements. The Hawaiian
survey consists of two profiles, one north of
Oahu and one north of Maro Reef (Figure 1). The
Reunion survey also consists of two profiles,
both north of Mauritius (Figure 2). These
high-resolution surveys provide evidence for
shallow fluid circulation and help to identify
its character at distance scales that are badly
aliased in the earlier coarse surveys and smoothed
out by averaging the results from multiple penetrations
at the local sites. Both surveys cross
archipelagic aprons characterized by a thick
wedge of volcaniclastic sediments deposited during
mass wasting events and which overlie pre-existing
sediments and oceanic basement. Root mean
square variations along the Oahu and Maro Reef
profiles are 15 and 5 mW m-2, respectively,
and along both Reunion heat flow profiles are
about 13 mW m-2 (Figures 1 and 2). These
heat flow profiles reveal greater scatter than
could plausibly be attributed to lateral or temporal
variations in mantle heat flux. The spectra
at both hotspots (Figure 3) are quite similar
with most of the signal in the heat flow occurring
at wavelengths less than a few hundred kilometers.
In each case there is a spectral peak at a wavelength
of the order of 10 km suggesting that the source
of perturbation is relatively shallow. Data
collected using the coarse survey strategy, at
intervals of 100 km or more, have aliased this
portion of the heat flow signal.
|
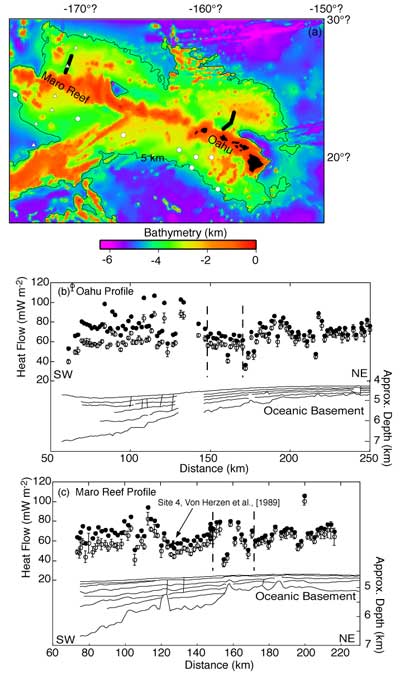
|
Figure 1. Thermal data from Hawaii. a) Location
of heat flow determinations. White symbols show location
of data from South Arch (circles) and Maro Reef (triangles)
(Von Herzen et al., 1982, 1989) and thick black lines
show data from Harris
et al. (2000a). The white triangle
colocated with the Maro Reef profile is site 4 of
Von Herzen et al., (1989). The extent of the Hawaiian
swell is approximated with the 5 km bathymetric contour.
Heat flow profile collocated with seismic reflection
line for offshore b) Oahu and c) Maro Reef. Heat
flow determinations uncorrected (open circles) and
corrected for the effects of sedimentation (solid
circles). Error bar represents standard deviation.
Line drawing of depth-converted seismic reflection
profile are shown in the lower portion of each panel
(Harris
et al., 2000a). Vertical dashed lines show
approximate divisions of Von Herzen (2004).
Likely candidates
for processes generating the observed variability
in the heat flow profiles are reviewed in Harris
et al. (2000a, 2000b). Variability
in the Hawaii profiles is greatest in the deepest
part of the flexural moat toward the volcanic
edifice, and in the Reunion profiles is greatest
near bare rock environments (Figures 1 and
2). Both of these observations seem more
consistent with buoyancy driven fluid flow
than recent volcanic intrusions. Harris
et al. (2000b) and Harris and McNutt (2007) argue that lateral fluid flow may mask anomalous
heat associated with the presence of a thermal
plume.
Because the scatter
in heat flow determinations is approximately
the same magnitude as predicted variations
in basal heat flow (e.g. Von Herzen et
al., 1989), our ability to resolve anomalous
basal heat flux will depend on a better understanding
of environmental conditions and non-conductive
processes. This understanding will likely require
more high-resolution heat flow surveys collocated
with seismic reflection lines, observations
of fluid flow, and more sophisticated modeling. Until
then, using heat flow data to distinguish between
thermal and non-thermal origins of midplate
swells may be premature.
|
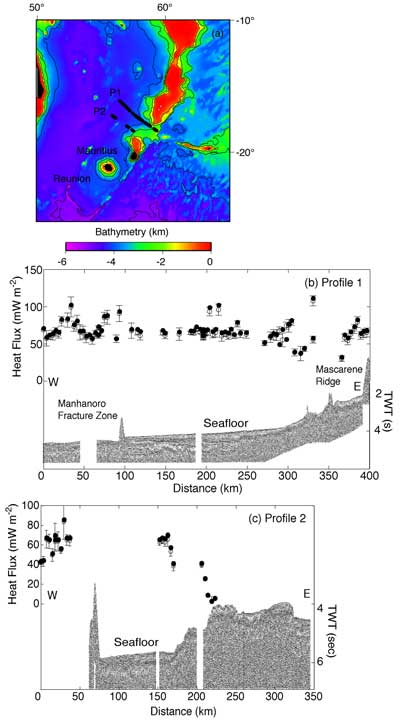
|
Figure 2. Thermal data from Reunion (Bonneville
et al., 1997). a) Location of heat flow determinations
at Reunion and heat flow determinations along b)
profile 1 and c) profile 2. Heat flow determinations
uncorrected (open circles) and corrected for the
effects of sedimentation (solid circles). Error bar
represents standard deviation. Seismic reflection
data is also shown.
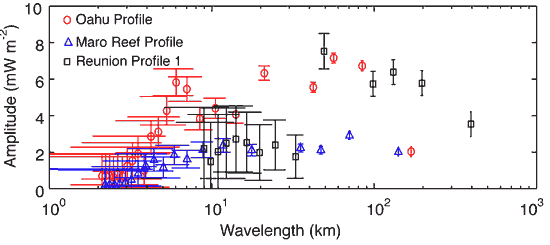
Figure 3. Spectral analysis
of closely spaced heat flow profiles on hotspot swells.
Vertical scale shows magnitude of harmonic coefficient
and uncertainty. Spectral smoothing was accomplished
through windowing four subsections of the original
series. Note spectral peaks of the order of 10 km.
Along the Oahu profile there is a strong peak at 7
km, along the Maro Reef profile there is a subtle peak
at approximately 10 km, and along Reunion profile 1
there is a peak at approximately 50 km.
References
-
-
Courtney, R. C. and M. Recq
(1986), Anomalous heat flow near the Crozet Plateau
and mantle convection, Earth
Planet. Sci. Lett.,
79, 373-384.
-
Courtney, R. C., and R. S. White
(1986), Anomalous heat flow and geoid across the
Cape Verde rise: Evidence for dynamic support
from a thermal plume in the mantle, Geophys.
J. Int., 87, 815-867.
-
Detrick, R. S., R. P. Von Herzen,
B. Parsons, D. Sandwell, and M. Dougherty (1986),
Heat flow observations on the Bermuda Rise and
thermal models of midplate swells, J. Geophys.
Res., 91, 3701-3723.
-
-
Harris, R. N., R. P. Von Herzen,
M. K. McNutt, G. Garven, and K. Jordahl (2000a),
Submarine hydrogeology of the Hawaiian archipelagic
apron, Part 1, Heat flow patterns north of Oahu
and Maro Reef, J. Geophys. Res., 105, 21,353-21,369.
-
Harris, R. N., G. Garven, J.
Georgen, M. K. McNutt, and R. P. Von Herzen (2000b),
Submarine hydrogeology of the Hawaiian archipelagic
apron, Part 2, Numerical simulations of coupled
heat transport and fluid flow, J. Geophys.
Res., 105, 21,371-21,385.
-
-
Stein, C. A., and S. Stein (1993),
Constraints of Pacific midplate swells from global
depth-age and heat flow-age models, The Mesozoic
Pacific, AGU Monog., 77, 53-76.
- Stein, C. A., and S. Stein
(2005), Why is heatflow not high at hotspots?,
http://www.mantleplumes.org/Heatflow.html.
-
Von Herzen, R. P. (2004), Geothermal
evidence for continuing hydrothermal circulation
in older (> 60 M.y.) ocean crust, E.E. Davis,
H. Elderfield (eds). in Hydrogeology of the
Oceanic Lithosphere, Cambridge Univ. Press,
414-447.
-
Von Herzen, R. P., R. S. Detrick,
S. T. Crough, D. Epp, and U. Fehn (1982), Thermal
origin of the Hawaiian Swell: Heat flow evidence
and thermal models, J. Geophys. Res., 87,
6711-6723.
-
Von Herzen, R. P., M. J. Cordery,
R. S. Detrick, and C. Fang (1989), Heat flow and
the thermal origin of hot spot swells: The
Hawaiian Swell revisited, J. Geophys. Res.,
94, 13,783-13,799.
last updated 4th
April, 2007 |