 |
Quartz
Mountain obsidian |
The
Oregon High Lava Plains: Proof against a plume
origin for Yellowstone?
|
|
|
Introduction
The High Lava Plains province (HLP) of central and
southeastern Oregon is an enigmatic volcanic province
characterized by bimodal volcanism with a west-younging
progression in ages of silicic volcanic rocks. Were
the age progression in a direction anywhere near the
back-azimuth of plate motion it would be widely interpreted
as the trace of a mantle plume. The age progression
crudely mirrors the northeast-younging trend of the
Yellowstone-Snake River Plain volcanic system (YSRP),
with both trends originating in a common region in
the axis of middle Miocene flood basalt volcanism
of the Steens and Columbia River Basalts (Figure 1).
Given these relations, the HLP, YSRP, and middle
Miocene flood basalts are commonly linked. The back-azimuth
of the age progression of the YSRP is in the direction
of North American plate motion, and therefore the
YSRP has generally been interpreted as a manifestation
of a hotspot (Armstrong et al., 1975; and
Yellowstone page),
widely presumed to be a mantle plume (e.g. Pearce
& Morgan, 1992; Smith & Braile,
1994). The middle Miocene flood basalts are commonly
interpreted as representing the plume-head phase of
this hypothesized mantle plume (Brandon &
Goles, 1988; Thompson & Gibson,
1991; Geist & Richards, 1993; Camp,
1994; Hooper, 1997; Camp et al.,
2003; and Columbia River Basalts
page). But how could the HLP possibly fit into a plume
model? The existence of the HLP trend is one of the
most frequently cited lines of evidence against the
plume model for the YSRP, e.g.:
Hamilton (1989), “Powerful evidence against
the notion of a Yellowstone hot spot is provided by
the presence in eastern Oregon of volcanic centers
by which magmatism progresses 200 km northwestward
during the past 10 m.y., a progression comparable
in time to that of the supposed Yellowstone hot spot
yet in an altogether different direction.”
I have been a part of an Oregon State University
research group, led by Anita Grunder, which has been
studying the High Lava Plains since the early 1990s.
Other researchers in this group include Martin Streck,
Jenda Johnson, Jim MacLean, Al Deino (Berkeley Geochronology
Center), Dave Graham, and Bob Duncan. In this review
I discuss the geology of the HLP, tectonic interpretations
of the HLP, and implications of these interpretations
for the plume model for Yellowstone.
|
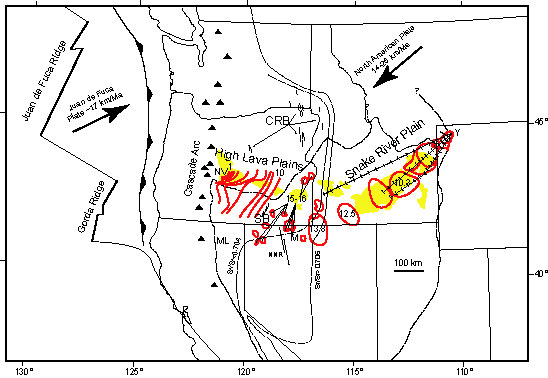
Figure 1
|
Geology and Petrology
of the High Lava Plains
The HLP is a bimodal volcanic province,
with only 8% of analyzed samples (n = 286 samples)
being intermediate in composition (this figure probably
over-representing the intermediate rocks because of
focused sampling due to their petrologic significance).
Basalts are mostly primitive, high-alumina, olivine
tholeiites (distinct from arc basalts with high-alumina
at low MgO). Trace element compositions of primitive
HLP basalts are similar to N-MORB, but with highly
elevated Ba, Sr, and Pb. Sr and Nd isotopic compositions.
HLP basalts are more evolved than MORB with a minimum
87Sr/86Sr of 0.7032. 3He/4He
ratios in HLP basalts range from 8.8 to 9.4 times
the atmospheric ratio (Jordan et al., 2000;
Graham et al., in prep).
Rhyolites were erupted in > 60
domes and dome complexes, three major ash flow tuffs
(Prater Creek, Devine Canyon, and Rattlesnake), and
several minor tuffs (Figure 2). Silicic rocks are
mainly high-silica rhyolites (> 75 wt% SiO2)
and are metaluminous to mildly peralkaline. (MacLean,1994;
Streck & Grunder, 1995; Johnson &
Grunder, 2000). Some rhyolites are isotopically
indistinguishable from associated basalts while others
are more isotopically evolved.
A systematic age progression of rhyolites
was recognized by MacLeod et al. (1975),
and recent 40Ar/39Ar geochronology
confirms this age progression (Jordan et al.,
in prep). Ages of rhyolite domes and tuffs decrease
westward from 10.5 Ma in the east to < 1 Ma in
the west. The distribution of age-progressive rhyolites
extends ~ 70 km south into the Basin and Range province,
though the rhyolite belt in the HLP is more robust
and continuous.
|
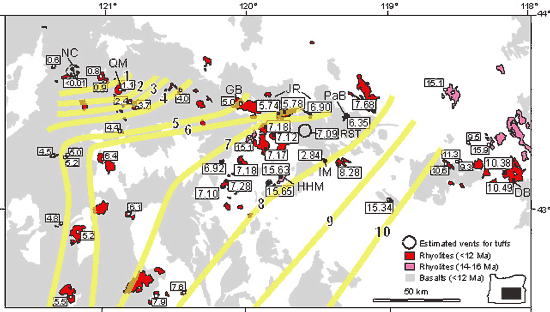
Figure 2
|
The age progression is commonly depicted
as a series of isochrons (Figure 2). These isochrons
are generally NE-trending. If propagation were perpendicular
to the isochrons, this geometry suggests NW-migration.
But from Duck Butte (DB in Figure 2) at 10.5 Ma in
the east, the belt of silicic magmatism, which coincides
with a belt of Pliocene and younger basaltic volcanism,
trends N75°W to the Newberry area. This observation
suggests that the NW-trend of the isochrons represents
an initial condition and that magmatism has migrated
westward as a “wave” rather than as a
point. The western isochrons become progressively
more closely spaced and E-trending. The spacing of
the isochrons indicates propagation rates of 35 km/m.y.
from 10 to 5 Ma and 15 km/m.y. since 5 Ma.
The present focus of this trend is
commonly interpreted to be Newberry volcano, however
Newberry is petrologically dissimilar to most HLP
volcanic centers and may represent more complicated
interactions between the HLP trend and the Cascade
arc. We, therefore, discourage the growing use of
the term “Newberry trend” or “Newberry
hotspot” to describe the HLP age progression,
preferring the antecedent “High Lava Plains
trend”.
Migrating silicic volcanism of the
HLP and northern Basin and Range is closely linked
with the distribution of closely-spaced NW-trending
normal faults of modest (< 100 m) offset, which
includes the Brothers fault zone.
|
Interpreting the
HLP: Implications for the plume model for Yellowstone
Several models have been put forth to explain the
HLP, several of which also seek to explain the YSRP:
-
-
Back-arc upwelling in response
to changing subduction geometry (Carlson &
Hart, 1987)
-
Entrainment of plume head material
in a subduction-induced mantle flow cell (Draper,
1991)
-
I comment on each of these models in order below.
|
(1) Preliminary results of GIS analysis of the time
space patterns of faulting across the HLP indicate
that extension on faults of the Brothers fault zone
has occurred at a rate of 0.2-0.5 %/m.y. across the
province since at least 7.6 Ma, and that faulting
did not propagate westward (ongoing research with
Soren Klingsporn, Whitman College). Therefore, the
propagating shear zones model is rejected for the
HLP. These faults may have played a role in allowing
magma to intrude and transit through the crust, but
can not explain mantle magma genesis (low rate of
extension, not adiabatic) or propagating crustal magmatism.
There is no evidence for a shear zone coincident with
the YSRP, and no need for one (extension north and
south of the Plain are of similar magnitude), so this
process doesn’t work there either.
(2) There is no evidence of slab steepening in this
time interval. Arc volcanism has occurred in the area
of the modern Cascade Range since ~ 40 Ma. Also, this
model envisioned high magnitudes of extension in the
back arc during the late Tertiary, and this is clearly
not the case (results described above).
|
(3) In explaining
the origin of the HLP, the model of Draper
(1991) invokes, rather than contradicts, the plume model
for the origin of Yellowstone. A shortcoming of this
model is that it is inconsistent with current models
of plume head dynamics in which a plume head flattens
as it approaches the lithosphere (Griffiths &
Campbell, 1991) and can be emplaced across a region
over 1,000 km across in less than a million years (Duncan
et al., 1997).
(4) The model of Humphreys
et al.
(2000) was created to demonstrate that a plume is
not necessary to explain either the HLP or YSRP trends,
though this model is consistent with the plume model
as well (a question-marked plume is, in fact, shown
in their cartoon). This model makes no attempt to explain
initial melting (middle Miocene) to create the residue
around which asthenosphere flows and wells up to generate
the trends. |
So models 1 and 2 are rejected and models 3 and 4
leave a plume origin permissible. To these I would
add two other plume-related mechanisms that could
explain west migrating silicic magmatism of the HLP:
(5) A plume head placed where global plate motion
models suggest it should be, back tracking from Yellowstone)
would have been emplaced east of the craton margin
in southern Idaho (Figure 1). Flow of plume head and
plume material on basal lithospheric topography (e.g.
Sleep, 1996) would have driven a westward
flow system. Additionally, this would explain the
focusing of middle Miocene flood basalt vents at the
craton margin!
(6) The plume head hypothesized in (5) would also
have emplaced more and hotter material in the east
(closer to the center) than the west. Therefore, the
incubation time for driving a crustal magmatic system
by advection and conduction would have been greater
in the west (further from center).
These models are depicted in Figure 3. A combination
of plume-lithosphere interaction and the model of
Humphreys
et al.
(2000) can explain all of the deviations of the
YSRP system from ideal mantle plume behavior. Some
critics of the plume model consider any modification
of the model from a vertical conduit arising from
the base of the mantle creating magmatism directly
above it to be ad hoc. I would argue that
the modifications described above are not ad hoc,
but based on physical processes that should be anticipated
in consideration of any mantle plume in a similar
setting (see Sleep, 2003 for well developed
arguments on this point).
|
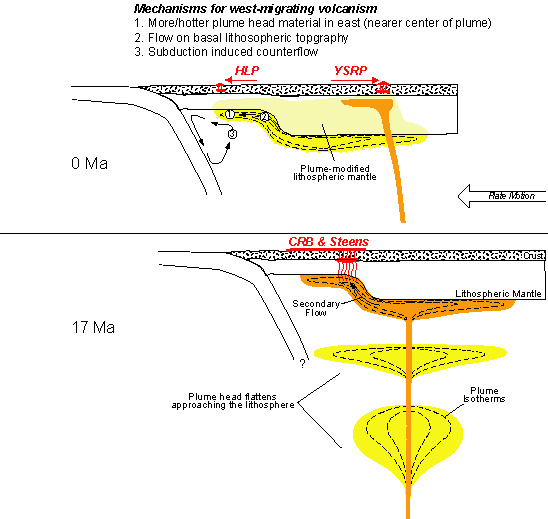
Figure 3
|
Are these models testable? A few
ideas come to mind. Past sublithospheric flow of plume
material might be detectible by appropriately aligned
shear wave anisotropy at some depth. The area of greatest
interest to me for this test would be the western Snake
River Plain, which connects the location where the hypothesized
plume should have been at 17 Ma (based on global plate
motion models) to where most of the middle Miocene flood
basalts erupted (NE Oregon, SW Washington). Sublithospheric
flow with some thermo-mechanical erosion of the overlying
lithosphere could be traced by the progressive introduction
of a radiogenic cratonic mantle component under the
accreted terranes to the west. Preliminary evidence
in support of this has been reported by Jordan et
al. (2002; and in prep.). |
Conclusion
Temporal, spatial, and petrologic relations
strongly suggest that the diverging age-progressive
volcanic trends of the Yellowstone-Snake River Plain
system, and the High Lava Plains province are linked.
That they be linked does not require that they be generated
by the exact same process as implied by those who argue
against a plume origin imply (see quote from Hamilton,
1989 above). Rather, it suggests that the processes
that formed them are probably linked.
The Yellowstone-Snake River Plain system
is widely considered to be the result of plate motion
over a mantle plume. Several reasonable physical mechanisms
are described above that might explain how plume-related
processes could also account for the High Lava Plains
trend. This is not to say that the plume
model has been proven, only that the existence of the
HLP trend does not disprove it.
Proof or disproof of the plume model
for Yellowstone should come from:
- high resolution tomographic studies of the Yellowstone
area that consider all physically reasonable potential
plume geometries; and
- resolution of the debate regarding the significance
of high 3He/4He ratios raised
by Anderson (e.g. 2000; and Helium
Fundamentals page). I am convinced that in order
to satisfy both geophysicists (who widely question
the significance of geochemical reasoning) and petrologists
(who commonly doubt the resolution and interpretation
of tomographic images) both issues must be resolved.
I, for one, will remain open to good
ideas from both sides of the plume debate for Yellowstone
and beyond. |
-
Anderson, D.L., 2000, The statistics
and distribution of helium in the mantle, International
Geology Review, 42, 289-311.
-
Armstrong, R.L., Leeman, W.P.,
and Malde, N.E., 1975, K-Ar dating, Quaternary and
Neogene rocks of the Snake River Plain, Idaho: American
Journal of Science, 275, 225-251.
-
Brandon, A.D., and Goles, G.G.,
1988, A Miocene subcontinental plume in the Pacific
Northwest: Geochemical evidence: Earth and Planetary
Science Letters, 88, 273-283.
-
Camp, V.E., 1994, Mid-Miocene
propagation of the Yellowstone mantle plume head
beneath the Columbia River basalt source region:
Geology, 23, 435-438.
-
Camp, V.E., Ross, M.E., Hanson,
W.E., 2003, Genesis of flood basalts and Basin and
Range volcanic rocks from Steens Mountain to the
Malheur River Gorge, Oregon: Geological Society
of America Bulletin, 115,
105-128.
-
Carlson, R.W., and Hart, W.K.,
1987, Crustal genesis on the Oregon Plateau: Journal
of Geophysical Research, 92,
6191-6206.
-
Christiansen, R.L., 1993, The
Yellowstone hot spot: Deep mantle plume or upper
mantle melting anomaly? [abstract]: EOS, Transactions
of the American Geophysical Union, 76,
602.
-
-
Draper, D.S., 1991, Late Cenozoic
bimodal magmatism in the northern Basin and Range
Province in southeastern Oregon: Journal of
Volcanology and Geothermal Research, 47,
299-328.
-
Duncan, R.A., and Richards, M.A.,
1991, Hotspots, mantle plumes, flood basalts, and
true polar wander: Reviews of Geophysics,
29, 31-50.
-
Duncan, R.A., Hooper, P.R., Rehacek,
J., Marsh, J.S., and A.R. Duncan, 1997, The timing
and duration of the Karoo igneous event, southern
Gondwana: Journal of Geophysical Research,
102, 18,127-18,138.
-
Geist, D., and Richards, M.,
1993, Origin of the Columbia Plateau and Snake River
Plain: Deflection of the Yellowstone plume: Geology,
21, 789-793.
-
Hamilton, W.B., 1989, Crustal
geologic processes of the United States, in Pakiser,
L.C., and Mooney, W.D., Geophysical framework
of the continental United States: Boulder, Colorado,
GSA Memoir 172, p. 743-781.
-
Hooper, P.R., 1997, The Columbia
River flood basalt province: Current status, in
Mahoney, J.J., and Coffin, M.F., eds., Large
igneous provinces: Continental, oceanic, and planetary
flood volcanism: American Geophysical Union
Geophysical Monograph 100, p. 1-27.
- Humphreys,
E.D., K.G. Dueker, D.L. Schutt, and R.B. Smith, Beneath
Yellowstone; evaluating plume and nonplume models
using teleseismic images of the upper mantle, GSA
Today, 10, 1-7, 2000.
-
Johnson, J. A., and Grunder,
A.L., 2000, The making of intermediate composition
magma in a bimodal suite: Duck Butte Eruptive Center,
Oregon, USA: Journal of Volcanology and Geothermal
Research, 95, 175-195.
-
Jordan, B.T., Graham, D.W., and
Grunder, A.L., 2000, Do helium isotopes in basalts
of the Oregon High Lava Plains implicate a Yellowstone
plume component? [abstract]: Geological Society
of America Abstracts with Programs, 32,(7),
p. 150
-
Jordan, B.T., Grunder, A.L.,
and Nelson, B.K., 2002, Basaltic volcanism of the
Oregon High Lava Plains [abstract]: Geological
Society of America Abstracts with Programs,
34, (5), p. 37.
-
MacLean, J.W., 1994, Geology
and Geochemistry of Juniper Ridge, Horsehead Mountain
and Burns Butte: implications for the petrogenesis
of silicic magmas on the High Lava Plains, southeastern
Oregon: M.S. thesis, Corvallis, Oregon State
University, 141 p.
-
MacLeod, N.S., Walker, G.W.,
and McKee, E.H., 1975, Geothermal significance of
eastward increase in age of Upper Cenozoic rhyolitic
domes in southeastern Oregon: U.S. Geological
Survey Open-File Report 75-348, 21 pp.
-
Pierce, K.L., and Morgan, L.A.,
1992, The track of the Yellowstone hotspot: Volcanism,
faulting, and uplift, in Link, P.K., Kuntz, M.A.,
and Platt, L.B., eds., Regional geology of eastern
Idaho and western Wyoming: Geological Society
of America Memoir 179, p. 1-53.
-
Sleep, N.H., 1996, Lateral flow
of hot plume material ponded at sublithospheric
depths: Journal of Geophysical Research,
101, 28,065-28,083.
-
Sleep, 2003, Mantle plumes?:
Astronomy and Geophysics, 44,
1.11-1.13.
-
Streck, M.J., and Grunder, A.L.,
1999, Enrichment of basalt and mixing of dacite
in the rootzone of a large rhyolite chamber: incluions
and pumices from the Rattlesnake Tuff, Oregon, Contributions
to Mineralogy and Petrology, 136,
193-21.
|
last updated 7th July, 2006 |
|
|