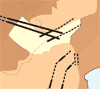 |
Basement
control on dyke distribution in Large Igneous Provinces:
Case study of the Karoo triple junction |
F.
Jourdana,b,c, G.
Férauda & H.
Bertrandb
aUMR-CNRS
6526 Géosciences Azur, Université de Nice-Sophia
Antipolis, O6108 Nice, France; Gilbert.FERAUD@unice.fr
bUMR-CNRS 5570, Ecole Normale Supérieure
de Lyon et Université Claude Bernard, 69364 Lyon,
France; herve.bertrand@ens-lyon.fr
cBerkeley Geochronology Center, 2455 Ridge
Road, Berkeley, CA 94709, USA; f.jourdan@curtin.edu.au
Click here to
download a PDF version of this webpage
Introduction
Continental flood basalts
(CFB) are huge magmatic events that are particularly
abundant during the Phanerozoic (for a review, see Courtillot
& Renne, 2001). Most of them are linked to
the Pangea mega-continent fragmentation (e.g.,
the Central Atlantic, Parana-Etendeka, Deccan), and
are characterized by giant dyke swarms emplaced in a
radial pattern (e.g., Ernst et al., 1995; Ed:
see also CAMP, Parana
and Deccan pages).
Dyke swarms are significant in part because their geometries
are generally considered to be paleo-stress and -strain
markers and as such they are useful for recognizing
proposed mantle plume impact sites (Ernst &
Buchan, 1997; Ed: see also Giant
dikes pages]. However, dyke swarms are also known
to be plate discontinuity markers (Fahrig er al.,
1986) and more recent investigations support the preponderant
role played by the pre-existing structure of the lithosphere
in controlling dyke-swarm emplacement (Mège
& Korme, 2003; Trumbull et al., 2004;
Jourdan
et al.,
2004) and/or CFB-related rift settings (Courtillot
et al., 1999; Tommasi & Vauchez, 2001).
Consequently, dyke distributions may not reflect a “primary”
structural signature (i.e. a mantle plume impact)
but could highlight preexisting lithospheric weaknesses.
Nevertheless, few studies have focused on this issue,
and the possible influence of various basement structures
(e.g., the edges of cratons, mobile belts,
shear zones, older dyke swarms) on CFB emplacement is
still poorly constrained. Here, we summarize our work
on the radiating dyke swarms of the Karoo large igneous
province. The results are derived mainly from papers
by Le Gall et al. (2002;
2005)
and Jourdan et al. (2004;
2005;
2006).
A
good example: the Karoo triple junction
The Karoo CFB formed during
a 185-177 Ma (Jourdan
et al.,
2005) magmatic event occurring prior to the southern
Gondwana break-up and the opening of the Indian Ocean.
It consists of tholeiitic lava-flows, sills and dykes
covering a paleo-surface in excess of 3x106
km2 (Eales et al., 1984). Huge dyke
swarms (the N110°-striking Okavango, the N70°-striking
Save-Limpopo, the knee-shaped Olifants River and the
N-S Lebombo dyke swarms; (Figure 1; e.g., Le
Gall et al.,
2002) appear to converge at the eastern edge of
the province defining a four-branch structure (the so-called
“triple-junction“; Figure1). Although the
Karoo “triple junction” has been regarded
as a Jurassic, CFB-related structure and a classical
example of a mantle plume impact site marker (e.g.,
Ernst & Buchan, 1997; 2003), it appears that:
- prior to our work the ages of the dyke
swarms were poorly constrained, and
- the field and petrographic evidence
contained in published geological maps suggests that
some of the dykes are pre-Karoo (Marsh, 2002).
One of the best tests to discriminate
between new and inherited dyke orientations is to detect
Precambrian dykes in the Jurassic swarms. Accordingly,
we efficiently distinguished between Jurassic and Precambrian
dykes using abbreviated, low resolution, 40Ar/39Ar
incremental heating schedules (the “speedy step-heating”
technique).
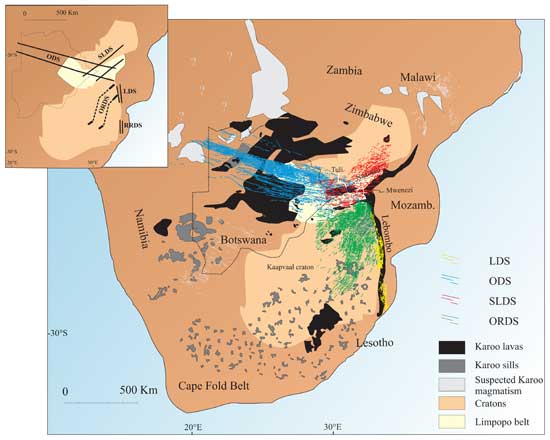
Figure
1: Location of the Karoo traps and regional dyke swarms;
dykes are based mostly on aeromagnetic data and follow
the compilation of Chavez Gomez (2001), modified by
Jourdan
et al. (2004). ODS: Okavango; ORDS: Olifants River;
SLDS: Save-Limpopo; LDS: Lebombo dyke swarms. Dotted
line corresponds to Botswana border. The Kaapvaal and
Zimbabwe cratons and Limpopo mobile belt are indicated.
Mozamb. = Mozambique. Inset represents schematic map
of the dyke swarms. Click here
or on figure for enlargement.
Results
and discussion: Inheritance of dyke orientations
40Ar/39Ar
“speedy” (Figure 2) and high-resolution
dating performed on dykes intruding either basement
or Karoo sequences, show that the Okavango dyke swarm
includes both Proterozoic (850-1700 Ma; n=8) and Jurassic
(179 ± 1 Ma; n=33) dykes (Figure 2). We obtained
similar results for the Save-Limpopo swarm that also
includes both Proterozoic (700-1700 Ma; n=14) and Jurassic
dykes (~179 Ma; n=8; Figure 2a). In addition, detailed
field observations (Figure 1; see zoomed areas in Jourdan
et al.,
2006) show evidence of basement influence via the
discernable rotation of these two swarms contiguous
with the Limpopo mobile belt architecture.
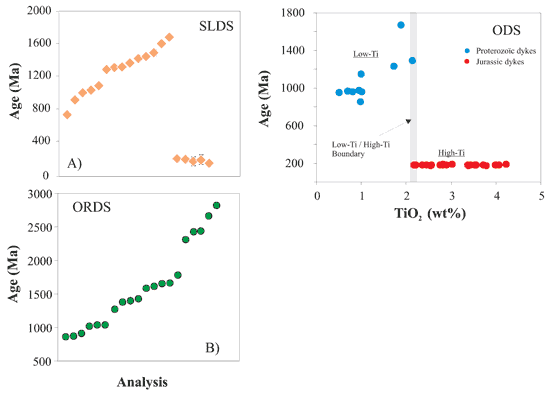
Figure
2: “Speedy step-heating” ages for the Okavango
(ODS; red dots) the Save-Limpopo (SLDS; orange diamonds),
and the Olifants River (ORDS; green dots) dyke swarms.
Note also the correlation between age (i.e. Proterozoic
or Jurassic) and the Ti-chemistry of the dykes. These
dates are low-resolution and should only be used to
differentiate between Jurassic, Proterozoic and possibly
Archaean dykes. Click here
or on figure for enlargement.
The Olifants River swarm
was investigated in its southern and northern regions.
The ages obtained seem to indicate two distinct Precambrian
dyke events, ranging from 850 to 1700 Ma and from 2500
to 2900 Ma. No ages consistent with the Karoo event
were obtained, although scarce Karoo dykes are probably
present in the Olifants River swarm. The Olifants River
dyke swarm can no longer be considered as a Karoo-aged
dyke swarm, confirming field observations previously
mentioned by Marsh (2002).
The last major branch
of the Karoo radiating swarm studied is the N-S Lebombo
dyke swarm which also includes the Rooi Rand dyke swarm
(e.g., Watkeys, 2002). Unfortunately, because
the number of outcropping dykes crosscutting the basement
is low, it is difficult to test the effect of inheritance
in this dyke swarm. Nevertheless, some N-S striking
dykes intrude the basement on the west side of the Lebombo
monocline and three of them have been dated between
1200 and 1650 Ma.
Broad-scale observations
show that the so-called “triple junction”
is a more complicated structure than portrayed in some
oversimplified sketch maps. First, the Okavango and
Save-Limpopo dyke swarms, though both of Karoo age (~179
Ma; Le
Gall et al.,
2002; Jourdan et al.
2004; 2005
and unpublished data) and referred to as a radiating,
do not radiate from Mwenezi area, but rather consist
of two crosscutting swarms (Wilson et al.,
1990; Ernst & Buchan, 1997; Figures 1 &
3) which partially overlap in the Tuli basin area (Figure
1). Secondly the Olifants River swarm does not converge
near Mwenezi but actually stops against the Lebombo
monocline hundreds of kilometers to the south (Figures
1 & 3).
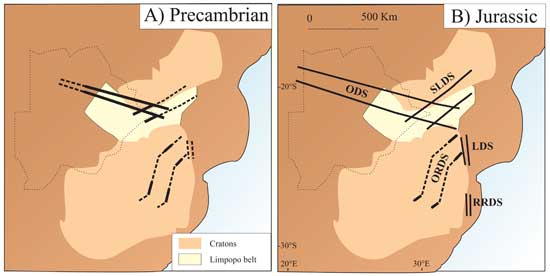
Figure
3: Sketch maps of the four radiating dyke swarms based
on 40Ar/39Ar ages, aeromagnetic
data, and field observations. Solid lines correspond
to segments of the swarms in which measurements and
observations have been made; dashed lines represent
inferred dyke swarm extensions. Densities of dykes within
the swarms are not shown. Plots show the diachronous
intrusion of dykes making up each swarm; dykes intruded
prior to the Karoo volcanism (A), and almost contemporaneously
with the ~180 Ma Karoo CFB (B). Click here
or on figure for enlargement.
Implications
for the plume model
Basement
control
The results suggest that
the “triple junction” structure is probably
an artefact characterized by:
-
strong basement
control,
-
the occurrence of
Precambrian dykes within at least two (and possibly
three) branches (Save-Limpopo and Okavango swarms
and possibly the Lebombo swarm),
-
a branch (Olifants
River) which is not Jurassic in age contrary to
previous assumptions (e.g., Ernst & Buchan,
1997; Reeves, 2000) and
-
a branch (the
Save-Limpopo) which does not really radiate from
the (Mwenezi area) focal point.
Therefore, the apparent
radiating structure is not sufficient to infer the existence
of a Jurassic mantle plume impact, because Proterozoic
dykes, which cannot be related to a plume impact in
Karoo time, define a similar pattern (Figure 3). It
is therefore likely that during Jurassic magmatism,
basement structures acted to control the orientation
of Karoo dykes. Thus, the Karoo dyke swarm geometry
should no longer be used to support the Karoo mantle
plume hypothesis; nevertheless, a mantle plume origin
for Karoo magmatism is not ruled out.
Other
evidence from 40Ar/39Ar geochronology
Additional 40Ar/39Ar
age data (Jourdan
et al.,
2005) demonstrate that the Karoo province was a
relatively long lasting event, including brief specific
magmatic events (e.g., Okavango dyke swarm).
Time-space emplacement of the Karoo basalts potentially
suggests an origin different from that of postulated
plume-related continental flood basalts such as the
Deccan or the Ethiopian-Yemen traps, for which emplacement
duration as short as ~1 Myr (Hofmann et al.,
2000) or ~1.5 Myr (Hofmann et al., 1997), respectively,
has been advocated. Such a long duration is more in
accordance with the time-span calculated by Turner
et al. (1996) for flood basalts produced by the
melting of a thick (>100 km thick) lithospheric mantle.
Implication
for other CFB-related dyke swarms
In this section,
we show that structural inheritance might have played
a significant role during the emplacement of the largest
Proterozoic and Phanerozoic (Gondwana related) flood
basalt dyke swarms.
Proterozoic
dyke swarms
The Mackenzie dyke
swarm (northwestern Canada), the largest radiating dyke
swarm on Earth, yielded U-Pb baddeleyite ages of 1267
± 2 Ma (Le Cheminant et al., 1989; 1991)
and is coeval with the Coppermine flood basalts and
the ultramafic Muskox intrusion (Ernst et al.,
1995). This NW-trending dyke swarm is flanked by a minor
and younger NW-trending Franklin dyke swarm dated by
U-Pb zircon/baddeleyite at 723 +4/–2 Ma (Heaman
et al., 1992). Although the Franklin dyke swarm
flanks the east of the Mackenzie swarm, the parallelism
of these two dyke swarms has been controlled by a single
crustal weak zone (Ernst et al., 1995).
Buchan et al.
(1993) reported geographically superposed dyke populations
in southern Canada yielding U-Pb baddeleyite ages of
2210 Ma (the Senneterre dyke swarm), 2167 Ma (the Biscotasing
dyke swarm) and 1140 Ma (the Abitibi dyke swarm). These
two examples illustrate that dyke swarm inheritance
might have played an important role in some Precambrian
dyke swarms.
Jurassic
dyke swarms
Here we provide
a brief overview of the relations between selected major
dyke swarms associated with Gondwana break-up (Figure
4) and their basement structures.
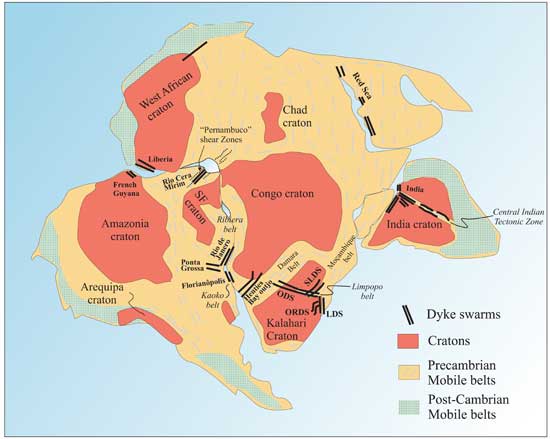
Figure 4: Schematic
map of part of the Gondwana continent before drifting
(modified after Schmitt et al., 2004) with superimposed
CFB-related Phanerozoic dyke swarms of various ages
(see text for references; the full extents of the CFB
are not represented). Cratonic Proterozoic blocks as
well as Pan-African / Braziliano, and Paleo-Proterozoic
Limpopo and Central Indian (Zhao et al., 2004) mobile
belts are shown. Click here
or on figure for enlargement.
Most dykes located
on the circum-Central Atlantic continents are related
to the ~200 Ma Central Atlantic Magmatic Province (e.g.,
Marzoli et al., 1999; Ed: See also CAMP
and Giant
dikes pages). Among these dykes, considered to converge
towards a focal zone around Florida (May, 1971),
one of the best defined swarms consists of WNW-ESE dykes
in Liberia and their counterparts in French Guyana.
The trend of these swarms follows the Pan-African belt
separating the West African and Amazonian cratons.
The South Atlantic
opening was preceded by intense magmatic activity at
~130 Ma forming the Parana-Etendeka CFB associated with
several major dyke swarms (Peate, 1997; Ed:
See also Parana page). Among
them, the most striking feature is the triple junction
formed by the Rio de Janeiro, Florianôpolis and
Ponta-Grossa dyke swarms in Brazil (Renne et al.,
1996; Deckart et al., 1998). The two first
branches apparently follow the Pan-African Ribiera and
Kaoko belt directions and the third branch follows the
Paleozoic Ponta Grossa arch (Chang et al.,
1992). To the north, the Rio-Cerà-Mirim dyke
swarm (Archanjo et al., 2000) is also Parana-related
(Marzoli et al., 2000) and extends on the northern
wedge of the Sao Francisco craton, in a direction parallel
to the Pan-African Pernambuco (and its African counterpart)
shear zones (Corsini et al., 1998) which may
have controlled the swarm orientation. Another Parana-Etendeka
related dyke swarm is the 100 km-wide Henties-Bay-Outjo
dyke swarm in Namibia (Trumbull et al., 2004)
which intrudes (and generally follows) the SW-NE oriented
Damara mobile belt. Interestingly, the dykes fan out
as they leave the Damara belt and intrude the Congo
craton at the easternmost extremity of the swarm (Figure
4) (Trumbull et al., 2004).
The gabbroic dyke
swarm of the Red Sea rift paralleling the rift structure
was emplaced between 24 and 21 Ma (Sebai et al.,
1991). No basement structural control is apparent for
the dyke orientations, but this swarm includes both
Neogene and Proterozoic dykes (H. Bertrand. &
G. Féraud, unpublished data), suggesting
that the dyking event associated with the Red Sea opening
followed an ancient Proterozoic direction.
Finally we mention
the radiating dyke swarm (e.g., Ernst et al.,
1995) related to the 65 Ma (Hofmann et al.,
2000) Deccan traps in India. One branch of the swarm
intrudes and follows the Central Indian Tectonic Zone
related to an Early Proterozoic collisional orogen between
the southern and northern Indian blocks (Zhao et
al., 2004; Ed: See also Deccan
pages). The two other branches are parallel to the western
edge of the Indian craton.
This brief overview
shows that the most prominent dyke swarms related to
Gondwana break-up (including those considered to define
several triple junctions) mainly follow craton boundaries
and are often parallel to mobile belts, shear zones
and other pre-existing basement structures (Figure 4).
The dykes were therefore possibly influenced by pre-existing
structures with regard to both location and orientation,
as we have shown in the Karoo case. Basement control
of CFB-related dyke swarms therefore casts doubt on
the “active role” of mantle plumes, if any,
in causing the “triple junction” pattern
marked by the occurrence of radiating dyke swarms. We
suggest that a careful re-examination at field scale
is required to better constrain the basement influence
on giant dyke swarm emplacement.
Conclusions
40Ar/39Ar
dating and structural analyses performed on the radiating
dyke swarms (and lava-flows) of the Karoo igneous province
suggest that:
-
Basement structures play a dominant (but not exclusive)
role in controlling the orientation of these major
dyke swarms.
-
The Precambrian Olifants River swarm is not of Karoo
age contrary to previous speculations.
-
The three major Karoo-age radiating dyke swarms
unambiguously include Proterozoic dykes indicating
a strong structural inheritance and precluding a
newly formed Jurassic radiating structure.
-
The apparent triple junction geometry was not induced
by the arrival of a deep mantle plume head but is
“inherited” from previous history of
the Kaapvaal and Zimbabwe cratons. Therefore, this
“triple junction” should no longer be
used as an argument for demonstrating (although
it does not exclude) the existence of a Karoo mantle
plume.
-
The “anomalously” long duration of the
Karoo province (~8 Myr) is also relevant to the
question of the existence of a mantle plume.
Consideration of
regional dyke swarms related to other Gondwana CFBs
shows that they too generally follow pre-existing lithospheric
structures such as craton boundaries, mobile belt orientations,
basement fabrics and major shear zones. We conclude
that the location and orientation of dyke swarms associated
with Gondwana break-up were generally controlled by
ancient basement structures and not by plume head impacts.
References
-
Archanjo,
C.J., Trindade, R.I, Macedo, J.W.P., Araujo, M.G.
(2000) Magnetic fabric of a basaltic dyke swarm
associated with Mesozoic rifting in northeastern
Brazil. J. South Am. Earth Sci. 13,
179-189.
-
Buchan,
K.L., Mortense, J.K., Card, K.D., (1993) North-east-trending
Early Proterozoic dykes of southern Superior Province:
multiple episodes of emplacement recognized from
integrated paleomagnetism and U-Pb geochronology,
Can. J. Earth Sci. 30,
1286-1296.
-
Chavez
Gomez S. (2001) A catalogue of dykes from aeromagnetic
surveys in eastern and southern Africa. ITC
publication number 80.
-
Corsini,
M., Lambert de Figueiredo, L., Caby, R., Feraud,
G., Ruffet, G., Vauchez, A. (1998) Thermal history
of the Pan-African/Brasiliano Borborema Province
of Northeast Brazil deduced from 40Ar/39Ar
analysis. Tectonophysics 285,
103-117.
-
Courtillot
V., Jaupart, C., Manighetti, I., Tapponnier, P.,
Besse. J. (1999) On causal links between flood basalts
and continental breakup. Earth Planet. Sci.
Lett. 166, 177-195.
-
Courtillot,
V.E., Renne, P.R., (2003) On the ages of flood basalt
events. C. R. Geosciences 335,
113-140.
-
Eales,
H.V., Marsh, J.S., Cox, K.G. (1984) The Karoo igneous
province: an introduction. In A.J. Erlank (Ed.),
Petrogenesis of the volcanic rocks of the Karoo
Province, Geological Society Special Publication
of South Africa 13, 1-26
-
Ernst
R.E., Head, J.W., Parfitt, E., Grosfils, E., Wilson.,
L. (1995) Giant radiating dyke swarms on Earth and
Venus. Earth-Sci. Rev. 39,
1-58.
-
Ernst
R.E., Buchan, K.L., Palmer, H.C. (1995) Giant dyke
swarms: characteristics, distribution and geotectonic
applications. In Physics and Chemistry of Dykes
(ed. A.H.G. Baer), pp. 3-21. Bakema Publication.
-
Ernst
R.E., Buchan, K.L. (2003) Recognizing mantle plumes
in the geological record. An. Rev. Earth Planet.
Sci. 31, 469-523.
-
Ernst,
R.E., Buchan K.L. (1997) Giant radiating dyke swarms:
their use in identifying pre-Mesozoic large igneous
provinces and mantle plumes. In J. Mahoney and M.
Coffin (Eds), Large Igneous Provinces: Continental,
Oceanic and Planetary Volcanism, AGU Geophysical
monograph, vol. 100 pp. 297-333
-
Fahrig,
W.F., Christie, K.W., Chown, E.H., Janes, D., Machado,
N. (1986) The tectonic significance of some basic
dyke swarms in the Canadian Superior Province with
special reference to the geochemistry and paleomagnetism
of the Mistassini swarm, Quebec, Canada Can.
J. Earth Sci. l.23, 238-253.
-
Heaman,
L.M. LeCheminant, A.N. Rainbird, R.H. (1992) Nature
and timing of Franklin igneous events, Canada: Implications
for a Late Proterozoic mantle plume and the break-up
of Laurentia, Earth Planet. Sci. Lett.
109, 117-131.
-
Hofmann
C., Courtillot, V. Féraud, G. Rochette, P.
Yirgu, G. Dtefo, E. Pick R. (1997) Timing of the
Ethiopian flood basalt event and implications for
plume birth and global change. Nature 389,
838-841.
-
Hofmann,
C., Féraud, G., Courtillot, V. (2000) 40Ar/39Ar
dating of mineral separates and whole rocks from
the Western Ghats lava pile: further constraints
on duration and age of the Deccan traps. Earth
Planet. Sci. Lett., 180, 13-27.
-
Jourdan,
F., Féraud, G., Bertrand, H., Kampunzu, A.B.,
Tshoso, G., Le Gall, B., Tiercelin, J.J. & Capiez
P. (2004). The Karoo triple junction questioned:
evidence from 40Ar/39Ar
Jurassic and Proterozoic ages and geochemistry of
the Okavango dyke swarm (Botswana). Earth Planet.
Sci. Lett. 222, 989-1006.
-
Jourdan
F., Féraud, G., Bertrand, H., Watkeys, M.K.,
Kampunzu, A.B., Le Gall, B. (2006) Basement control
on dyke distribution in Large Igneous Provinces:
Case study of the Karoo triple junction. Earth
Planet. Sci. Lett. 241, 307-322.
-
Jourdan
F., Féraud, G., Bertrand, H., Kampunzu, A.B.,
Tshoso, G. & Le Gall, B. (2005) The Karoo Large
Igneous Province: brevity, origin and relation with
mass extinction in question from new 40Ar/39Ar
age data, Geology 33,
745-748.
-
Le
Cheminant, A.N., Heaman, L.M. (1989) Mackenzie igneous
events, Canada: Middle Proterozoic hotspot magmatism
associated with ocean opening, Earth Planet.
Sci. Lett. 96, 38-48.
-
Le
Cheminant, A.N., Heaman, L.M. (1991) U-Pb ages for
the 1.27 Ga Mackenzie igneous events, Canada: Support
for a plume initiation model, Program with Abstracts,
Geol. Assoc. Can. 16,
(A73).
-
Le
Gall B., Tshoso, G., Jourdan, F., Féraud,
G., Bertrand, H., Tiercelin, J.J., Kampunzu, A.B.
Modisi, M.P., Dyment, M., Maia J. (2002) 40Ar/39Ar
geochronology and structural data from the giant
Okavango and related mafic dyke swarms, Karoo igneous
province, Botswana. Earth Planet. Sci. Lett.
202, 595-606.
-
Le
Gall, B., Tshoso, G., Dyment, J., Kampunzu, A.B.,
Jourdan, F., Féraud, G., Bertrand, H., Aubourg,
C., Vétel V. (2005) Structure of the Okavango
giant mafic dyke swarm (NE Botswana) and its significance
within the Karoo large igneous province of southern
Africa, J. struct. Geol. 27,
2234-2255.
-
Marsh
J.S. (2002) "The geophysical mapping of Mesozoic
dyke swarms in southern Africa and their origin
in the disruption of Gondwana, J. Afr. Earth
Sci. 30 (2000) 499–513"
: DISCUSSION. J. Af. Earth Sci. 35,
525-527.
-
Marzoli,
A., Renne, P., Piccirillo, E., Ernesto, M., Bellieni,
G., De Min. A. (1999) Extensive 200-million-year-old
continental flood basalts of the Central Atlantic
Magmatic Province. Science 284,
616-618.
-
Marzoli,
A., Renne, P.R., Piccirillo, E.M. (2000) Ar/Ar geochronology
of Mesozoic continental basaltic magmatism and the
opening of the central, equatorial and southern
Atlantic ocean. In: Penrose 2000: Volcanic Rifted
Margins. Geology department, Royal Holloway, University
of London, p. 54.
-
May,
P.R. (1971). Patterns of Triassic diabase dikes
around the north Atlantic in context of predrift
position of the continents. Geol. Soc. Am. Bull.
82, 1285-1292.
-
Mège
D., Korme, T. (2004) Dyke swarm emplacement in the
Ethiopian Large Igneous Province: not only a matter
of stress. J. Vol. Geotherm. Res. 132,
283-310.
-
D.W.
Peate, The Parana-Etendeka Province. (1997) In J.J.
Mahoney, M.F. Coffin, Large igneous provinces;
continental, oceanic, and planetary flood volcanism,
AGU Geophysical Monograph Vol. 100, pp. 217-245
-
Reeves
C. (2000) The geophysical mapping of Mesozoic dyke
swarms in southern Africa and their origin in the
disruption of Gondwana. J. Afr. Earth Sci.
30, 499-513.
-
Schmitt,
R.S., Trouw, R.A., Schmus, J.W.R., Pimentel, M.M.
(2004) Late amalgamation in the central part of
West Gondwana: new geochronological data and the
characterization of a Cambrian collisional orogeny
in the Ribeira Belt (SE Brazil). Precambrian
Res. 133, 29-61.
-
Sebai,
A., Zumbo, V., Féraud, G., Bertrand, H.,
Hussain, A.G., Giannérini G., and Campredon,
R. (1991) 40Ar/39Ar dating of alkaline and tholeiitic
magmatism of Saudi Arabia related to the early red
Sea rifting, Earth Planet. Sci. Lett. 104,
473-487.
-
Tommasi
A., Vauchez., A. (2001) Continental rifting parallel
to ancient collisional belts: an effect of the mechanical
anisotropy of the lithospheric mantle. Earth
Planet. Sci. Lett. 185, 199-210.
-
Trumbull
R.B., Vietor, T., Hahne, K., Wackerle, R., Ledru,
P. (2004) Aeromagnetic mapping and reconnaissance
geochemistry of the Early Cretaceous Henties Bay
Outjo dike swarm, Etendeka Igneous Province, Namibia.
J. Afr. Earth Sci. 40,
17-29.
-
Turner,
S., Hawkesworth, C., Gallagher, K., Stewart, K.,
Peate, D., and Mantovani, M., (1996) Mantle plumes,
flood basalts and thermal models for melt generation
beneath continents: Assessment of a conductive heating
model and application to the Parana. J. Geophy.
Res. 101, 11,503–11,518
-
Watkeys
M.K. (2002) Development of the Lebombo rifted volcanic
margin of southeast Africa. In Volcanic rifted
margin, Vol. 362 (eds. M. A. Menzies, S.L.
Klemperer, C.J. Ebinger, J. Baker), pp. 29-48. Geol.
Soc. Am. Spec. Pap.
-
J.F.
Wilson, (1990) A craton and its cracks; some of
the behaviour of the Zimbabwe Block from the late
Archaean to the Mesozoic in response to horizontal
movements, and the significance of some of its mafic
dyke fracture patterns, J. Afr. Earth Sci.
10, 483-501.
-
Zhao,
G., Sun, M., Wilde, S.A., Li, S. (2004) A Paleo-Mesoproterozoic
supercontinent: assembly, growth and breakup. Earth-Sci.
Rev. 67, 1-158.
last updated 15th
February, 2006 |