Introduction
Current debates
on the existence of mantle plumes originate substantially
from the interpretations of supposed signatures of plume-induced
surface topography that are compared with the predictions
of conventional fluid-dynamic models of plume-lithosphere
interactions. However, conventional models are poorly
suited to predict surface evolution, specifically in
case of continental lithosphere. In general, the boundary
conditions imposed in such models correspond to a fixed
upper surface, and the lithosphere is represented by
a simple viscous mono-layer with a rigid top. More realistically,
the plate surface is free and its deformation is controlled
by the elastic–brittle–ductile rheological
properties and the contrasting layered structure of
thick continental lithosphere. Effective surface topographical
evolution computed from conventional models thus provides
only a rough estimate based on simple isostatic assumptions
(Figure 1).
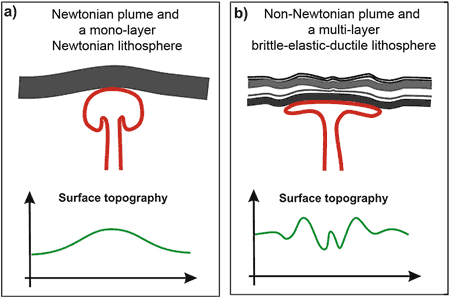
Figure 1. Sketches
of plume-lithosphere interactions: (a) a “conventional”
plume impinges on a single-layer viscous lithosphere
resulting in a single, large-wavelength topographic
signature ; (b) when realistic lithosphere rheology
(brittle – elastic –ductile) and multi-layer
structure are considered, several wavelengths of surface
topographic undulations are expected.
Modelling
In order to make
more meaningful comparisions of observations and models,
we introduce a thermo-mechanical model of plume-lithosphere
interaction that incorporates “realistic”
continental lithosphere. This model includes: (1) a
natural free-surface boundary condition, (2) an explicit
elastic–viscous–plastic (EVP) rheology,
and (3) a stratified continental lithosphere structure.
Our numerical experiments
assume a non-Newtonian mantle plume that initiated at
a depth of 650 km. The plume rises to the base of a
stratified brittle–elastic–ductile continental
lithosphere. The vertical rheological profile and the
structure of the lithosphere are varied from “young
juvenile” lithosphere to “old, craton-like”
lithosphere. A separate set of experiments was conducted
to test the influence of lateral variations in lithospheric
structure such as inherited blocks, cratons and intraplate
boundaries.
The surface amplitude
and wavelength predicted by our experiments differ from
the predictions of conventional models (Figure 2):
- the amplitudes
of the predicted surface uplift are reduced by as
much as 50%;
- the initial largest
wavelength of uplift is 20-30% greater;
- there are additionally
prominent secondary harmonics of surface deformation
that correspond to small “tectonic” wavelengths
(from 50-100 km to 300-500 km).
The thick continental
lithosphere thus works as a frequency modulator of the
plume impact. Due to its flexural resistance it increases
the largest wavelengths produced by the plume head and
generates smaller surface wavelengths associated with
crust-mantle decoupling and intraplate instabilities.
Attenuation of long surface wavelengths (> 500 km)
occurs in the case where weak, ductile lower crust separates
the upper crust from the mantle and accommodates the
mantle domal uplift produced by the plume. The initial
surface reaction to the plume ascent is a regional domal
uplift. However, in most cases it is followed by surface
subsidence (Figure 2) resulting from differential crustal
thinning produced both by the mantle uplift and by gravity-driven
flow in the lower crust. The mantle uplift may be rapidly
suppressed by negative Rayleigh-Taylor instabilities
that develop in the mantle lithosphere. For these reasons,
plumes may not always produce detectable large-scale
topography. Instead, they produce easily detectable
alternating, small- or medium-scale features normally
attributed to regional tectonics. Large-scale, mono-harmonic
deformation, predicted by conventional models, develops
only in the case of strong oceanic or very cold, thick
continental lithosphere.
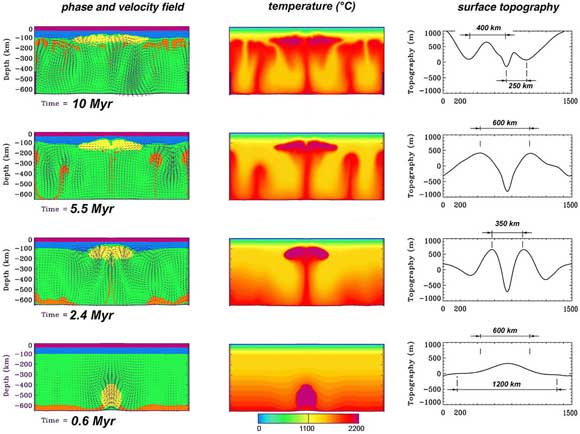
Figure 2. Results of
numerical experiments on plume-lithosphere interaction
beneath a “realistic” continental lithosphere.
The plume Rayleigh number is 106. Surface
topography shows three types of signature at different
phases of evolution. A single topography wavelength
is only observed when the plume head is still en route
to the bottom of the lithosphere. When the plume head
impinges on the base of the lithosphere, surface subsidence
is observed above the center of the plume head, as well
as above each of its edges (case at 2.4 Myr). The final
stages show more irregular topographic signatures, while
the initial plume head is flattened and secondary plumes
have ascended. Click
here for enlargement.
Results
As can be seen,
the important differences from the predictions of conventional
models are related to plate bending, mechanical decoupling
of crustal and mantle layers and tension-compression
instabilities that produce transient topographic signatures
such as uplift and subsidence at a large (> 500 km)
and small scales (300-400 km, 200-300 km and 50-100
km). Four main differences from the conventional viscous
models are noteworthy (Figure 2):
-
the
appearance of additional short- and medium-intermediate
wavelengths of deformation (alternating basins and
uplifts), which do not necessarily correlate with
the size of the plume head;
-
poly-phase
deformation related to visco-elastic relaxation
and progressive reduction of the resistance of lithospheric
layers as the plume head flattens horizontally;
-
reduced
impact of plume ascent on the amplitude of surface
elevations; and
-
a
concentration of plume-related extension in the
mantle lithosphere, with little effect on the decoupled
crust; in the case of strong mechanical decoupling
the crust may not even “feel” the plume.
Experiments also
suggest that the plate surface reacts to the plume ascent
well before its final emplacement.
The basin-scale uplifts
and subsidences result from several processes:
- interplay between the rheological layers
within the lithosphere;
- visco-elastic relaxation (Maxwell time
for the lithosphere may reach 10 Myr);
- plume-induced Rayleigh-Taylor instabilities
in the mantle.
These small-scale undulations
are superimposed on lithospheric-scale wavelengths,
as it can be verified in east Africa (Figure 3a), where
an underlying mantle plume would behave differently
in the northern and southern parts (the Main Ethiopian
rift and the Tanzania areas). Spectral analyses of surface
topography in east Africa show two dominant wavelengths
(at 200-400 km and 30-80 km), reflecting lithospheric
and crustal scale instabilities. Similar analyses on
numerical topography confirm this bimodal character
(Figure 3b).
a)
Data profiles
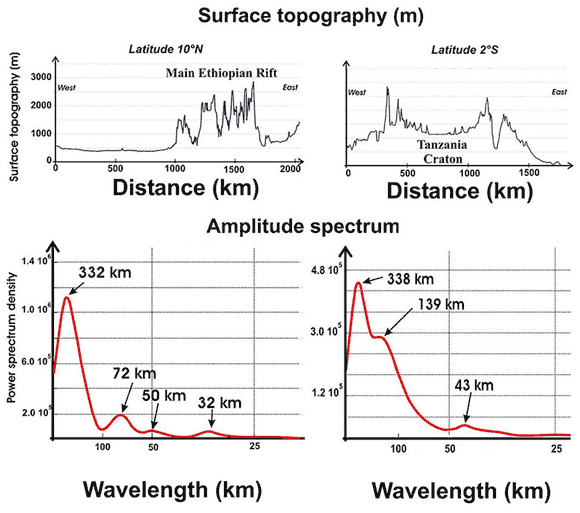
b)
Model predictions
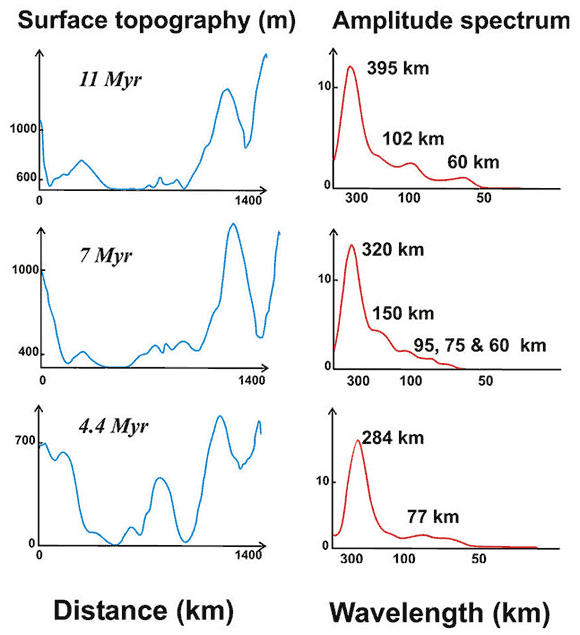
Figure 3. Comparison
of a) real topography and amplitude spectra profiles
across the Ethiopian rift and Tanzanian craton (Africa),
with b) topography and amplitude spectra predicted by
the model. The model reproduces the same three characteristic
wavelengths (approx. 300, 100 and 50 km) as observed.
The
instabilities that develop at the top and the sides
of the plume head provide a mechanism for crustal delamination.
In the case of a plume rising below “normal”
lithosphere bounding a craton, there is important lateral
flow of material from the plume head to the base of
the craton. This suggests a new mechanism for crustal
growth, in which surface magmatism is not required.
Lithospheric faulting at the craton edges and the enhanced
magmatic activity can explain such geological events
as apparent plume-related metallogenic crises in Archeen
West Africa and Australia.
The
continental Moho presents a strong density and rheology
barrier to upward propagation of plume material. The
role of the competent mechanical “core”
of the crust may be as important as that of the mantle
lithosphere, particularly for early Archean lithosphere
or lithosphere younger than 300 Ma. Thus, crustal deformation
interferes with mantle deformation resulting in complex
periodic subsidence/uplift patterns. Surface evolution
often begins with a central uplift, followed by subsidence
accompanied by formation of series of smaller basins.
Closing
remarks
The new EVP model
infers that the plume model can explain complex “tectonic”
processes such as polyphase rifting, lower-crustal delamination,
large-scale faulting, crustal growth, etc. Distinct
topographic wavelengths or temporally spaced events
observed, for example, in the East African Rift system,
as well as over the French Massif Central and the Pannonian-Carpathian
system (Ed: see also Carpathians
page), can be explained by a single plume impinging
at the base of the continental lithosphere, i.e.
without the necessity of evoking any complex asthenospheric
upwelling or additional tectonic events. Plume-lithosphere
interactions can explain a number of other key phenomena
such as the simultaneous occurrence of a climax of extension
of young plates/segments and a climax of compression
in surrounding belts. This scenario is consistent with
differential mantle lithosphere thinning of the younger
plate and is compatible with inferences from seismic
tomography.
|