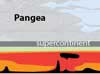 |
A plumeless supercontinent has more panache |
Jérôme Ganne
Géosciences Environnement Toulouse, UMR 5563 CNRS / UR 234 IRD / UPS, 14, avenue Édouard Belin, 31400 Toulouse, France, jerome.ganne@Get.omp.eu
This webpage is a summary of: Ganne, J., Feng, X., Rey, P. F., De Andrade, V. (2016). Statistical petrology reveals a link between supercontinents cycle and mantle global climate. American Mineralogist.
Supplementary material
The aggregation and dispersal of supercontinents control Earth’s geological history and influence climate and biological evolution. But what is the relationship of these supercontinents to the deep interior? A study published in the journal American Mineralogist, involving a team of researchers from the University of Toulouse (France) and Earthbyte’s Basin Genesis Hub at the University of Sydney, presents a new global perspective of the magmatic history of Pangea, the latest supercontinent.
Over the past century the study of volcanic rocks has provided a vast amount of data which have been used in all branches of Earth Sciences (Bowen, 1928). Numerous interpretations of these data have been proposed and used to develop evolutionary models that shape our understanding of global geodynamics. At the core of geochemical geodynamics is the concept of ‘hot spots’, the surface expression of mantle plumes (Morgan, 1971). These are proposed to account for many observations including volcanic island chains in the oceans, the dynamic uplift of oceanic lithosphere, basaltic dyke swarms in the continents, and large continental volcanic provinces (Ernst & Buchan, 2001). In the Wilson cycle, which describes the formation and destruction of oceanic lithospheres and the episodic formation of supercontinents, mantle plumes are widely considered to be influential in the fracturing of supercontinents (Morgan, 1983; Courtillot, 1999; Storey et al., 2001).
The proposal that supercontinents could act as blankets, warming up the mantle underneath and leading to intraplate magmatism (Anderson, 1982), challenges the plume paradigm. According to this hypothesis, there is no need to invoke deep mantle instability to produce thermal anomalies at Earth’s surface. Although many numerical studies have strengthened this hypothesis (e.g., Gurnis, 1988; Grigné et al., 2005; Coltice et al., 2007; Lénardic et al., 2011) no petrological data have been presented in support of supercontinent mantle warming. This is unsurprising because of the scale problem–plate-scale thermal processes have to be linked to petrological studies on the scale of individual volcanic centres. How can we reconcile data gathered on these different scales? Which petrological information from which rock samples should we focus on and, importantly, how can we link this information to mantle geodynamics?
The petrological data extracted from rocks in hand specimen are rarely faithful representations of larger petrological systems. Many variables, from the size of the sample to the analytical method and the instrument used to perform the measurements, contribute to the total error (Ganne et al., 2012). In addition, many geological processes conspire to obscure the original geochemical signal. The emerging field of geostatistics (Keller & Schoene, 2012) provides tools to deal with these uncertainties by assessing the trend of averaged values in large, multi-scale data sets. In order to track the temperature of mantle-derived magmas through time the research team extracted, from the Georoc database, over 16 million metadata including samples’ bulk composition and mineralogical composition.
The final statistical analysis shows that over the past 600 Ma, the average temperature of continental magmas increased before decreasing by up to 100°C (Figure 1). The warming period is coeval with the aggregation of Pangea from 600 to 200 Ma, whereas the progressive cooling is synchronous with Pangea’s dispersal from 200 Ma onward. This confirms that a link exists between the temperature of the sub-continental mantle and the supercontinent cycle. It further suggests that magmas originating at shallow levels beneath continents are probably linked to tectonic processes, rather than global mantle convection, in particular at times of major plate re-organization (Husson & Conrad, 2012).
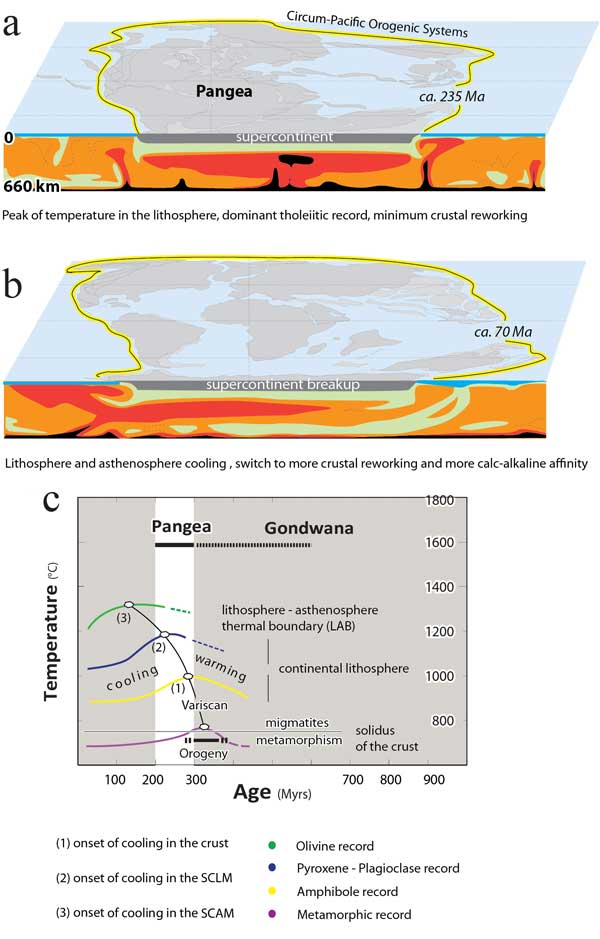
Figure 1: Supercontinent cycle and thermal regime. (a, b) Sketches illustrating plate and mantle dynamics and climate (Farrington et al., 2010) during amalgamation and breakup of the Pangea supercontinent. Paleogeographic configurations are based on Triassic and Cretaceous reconstructions (Veevers, 2004). The external (circum-Pacific) system comprises a number of discrete orogens that together have probably existed for 550 Ma (Collins et al., 2011). (c) Thermal peaks for magmatic pyroxenes and plagioclases (~225 Ma; blue curve), dominantly tapping (or considered to reflect conditions in) the sub-continental lithospheric mantle, span a period of orogenic collapse for the belts suturing the Pangea supercontinent. Thermal peak for olivines (~125 Ma; green curve), dominantly tapping the sub-continental asthenospheric mantle, corresponds to a period of enhanced supercontinent breakup.
This work introduces a suite of new questions and predictions regarding the formation of continental margins and their associated sedimentary basins. In particular, it predicts that continental margins formed during the dispersal of supercontinents evolved over warmer, more buoyant mantle. This may have implications regarding the involvement of magmatic input into continental margins as well as the stratigraphy of sedimentary basins through uplift of the margin and subsidence history.
References
-
Anderson, D. L. (1982). Hotspots, polar wander, Mesozoic convection and the geoid. Nature, 297, 391-393.
-
Bowen, N.L. (1928). The Evolution of the Igneous Rocks. Princeton University Press, Princeton, New Jersey.
-
Collins, W.J., Belousova, E.A., Kemp, A.I.S., and Murphy, J.B. (2011) Two contrasting Phanerozoic orogenic systems revealed by hafnium isotope data. Nature Geoscience, 4, 334-337.
-
Coltice, N., Phillips, B.R., Bertrand, H., Ricard, Y. and. Rey, P. (2007). Global warming of the mantle at the origin of flood basalts over supercontinents. Geology, 35, 391-394.
-
Courtillot, V., Jaupart, C., Manighetti, I., Tapponnier, P., and Besse, J. (1999). On causal links between flood basalts and continental breakup: Earth and Planetary Science Letters, 166, 17-195.
-
Ernst, R.E., Buchan, K.L. (2001). Large mafic magmatic events through time and links to mantle-plume heads. In: Ernst, R.E., Buchan, K.L. (Eds.), Mantle Plumes: Their Identification Through Time. Special Paper-Geological Society of America, 352, 483-566.
-
Farrington, R.J., Stegman, D.R., Moresi, L.N. Sandiford, M., and May, D.A. (2010) Interactions of 3D mantle flow and continental lithosphere near passive margins. Tectonophysics, 483, 20-28.
-
Ganne, J. et al. (2012). Modern-style plate subduction preserved in the Palaeoproterozoic West African Craton. Nature Geoscience, 5, 60-65.
-
Grigné C., Labrosse S., and Tackley P.J. (2005). Convective heat transfer as a function of wavelength: Implications for the cooling of the Earth. Journal of Geophysical Research, 110, B03409.
-
Gurnis, M. (1988). Large-scale mantle convection and the aggregation and dispersal of supercontinents. Nature, 332, 695-699.
-
Husson, L. & Conrad, C.P. (2012). On the location of hotspots in the framework of mantle convection. Geophysical Research Letters, 39, L17304.
-
Keller, C.B. and Schoene, B. (2012). Statistical geochemistry reveals disruption in secular lithospheric evolution about 2.5 Gyr ago. Nature, 485, 490-493.
-
Lenardic, A., Moresi, L., Jellinek, A. M., O'Neill, C. J., Cooper, C. M. and Lee, C.-T. A. (2011). Continents, supercontinents, mantle thermal mixing, and mantle thermal isolation: Theory, numerical simulations, and laboratory experiments. Geochemistry Geophysics Geosystems, 12.
-
Morgan, W.J. (1983). Hotspot tracks and the early rifting of the Atlantic: Tectonophysics, 94, 123-139.
-
Storey, B.C., Leat, P.T., Ferris, J.K. The location of mantle plume centers during the initial stages of Gondwana breakup In: Ernst, R.E., Buchan, K.L. (Eds.), Mantle Plumes: Their Identification Through Time. Special Paper-Geological Society of America, 352, 71-80 (2001).
-
Veevers, J.J. (2004) Gondwanaland from 650–500 Ma assembly through 320 Ma mergers in Pangea to 185–100 Ma breakup: supercontinental tectonics via stratigraphy and radiometric dating. Earth-Science Reviews, 68, 1-132.
last updated 9th December, 2016 |