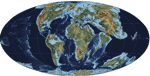 |
The relationship between Pangaea breakup and Large Igneous Provinces |
Alexander L. Peace1, Jordan J.J. Phethean2, Dieter Franke3, Gillian R. Foulger4, Christian Schiffer5, J. Kim Welford6, Greg McHone7, Sergio Rocchi8, Michael Schnabel3 & Anthony G. Doré1
1School of Geography and Earth Sciences, McMaster University, 1280 Main Street West, Hamilton, Ontario, Canada L8S 4K1
2School of Environmental Sciences, University of Derby, Kedleston Road, Derby, UK
3Bundesanstalt für Geowissenschaften und Rohstoffe (Federal Institute for Geosciences and Natural Resources), Germany
4Department of Earth Sciences, Durham University, Durham, UK
5Department of Earth Sciences, Uppsala University, Villavägen 16, 75236 Uppsala, Sweden
6Department of Earth Sciences, Memorial University of Newfoundland, St. John’s, Newfoundland, Canada
7Dexters Lane, Grand Manan, New Brunswick E5G3A6, Canada
8Dipartimento di Scienze della Terra, Università di Pisa, Pisa, Italy
This webpage is a summary of Peace, A.L., Phethean, J.J.J., Franke, D., Foulger, G.R., Schiffer, C., Welford, J.K., McHone, G., Rocchi, S., Schnabel, M., Doré, A.G., 2019. A review of Pangaea dispersal and Large Igneous Provinces – In search of a causative mechanism. Earth-Science Rev. in press. https://doi.org/10.1016/j.earscirev.2019.102902
Introduction: The supercontinent cycle and overview of Pangaea dispersal
Throughout geological time the majority of continental lithosphere has several times assembled into ‘supercontinents’ that subsequently dispersed (Pastor-Galán et al., 2018). The latest supercontinent, Pangaea (Figure 1), was mostly assembled by 320 Ma. It disintegrated throughout the Mesozoic with the final fragmentation forming the North Atlantic Ocean at about 55 Ma (Frizon De Lamotte et al., 2015; Hansen et al., 2009; Stampfli et al., 2013).
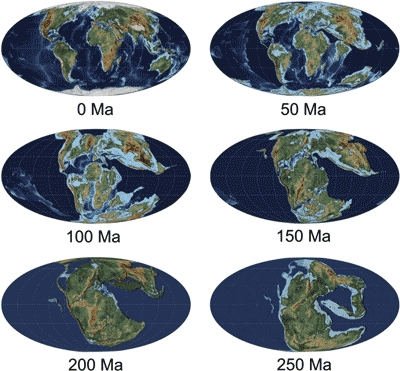
Figure 1. Disintegration of Pangaea from 250 Ma – present (e.g., Frizon De Lamotte et al., 2015) using the palaeogeographic reconstruction PALEOMAP PaleoAtlas for GPlates (Scotese, 2016).
The dispersal of Pangaea into its constituent continents was accompanied by extensive, episodic magmatic activity. Several Large Igneous Provinces (LIPs) formed including the Central Atlantic Magmatic Province (CAMP) and the North Atlantic Igneous Province (NAIP).
In our recent article (Peace et al., 2019) we reviewed the chronology of Pangaea breakup and LIP formation to test the hypothesis that the arrival of plume heads initiates continental breakup. Specifically, we reviewed:
- Triassic formation of the Central Atlantic Ocean,
- Middle Jurassic breakup between East and West Gondwana,
- Early Cretaceous opening of the South Atlantic, and
- Mesozoic-Cenozoic formation of the North Atlantic.
1. Triassic formation of the Central Atlantic Ocean
The Central Atlantic comprises the oldest part of the Atlantic Ocean, with oceanic crust dating back to the Triassic-Jurassic boundary (Biari et al., 2017) (Figure 2). Breakup of the Central Atlantic was contemporaneous with emplacement of the Central Atlantic Magmatic Province (CAMP) LIP, which has been linked with the end-Triassic mass extinction (Marzoli et al., 1999).
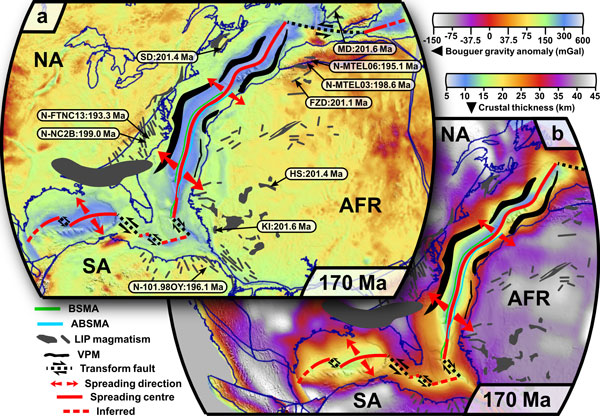
Figure 2: Breakup of the Central Atlantic at 170 Ma. a) Reconstructed Bouguer gravity anomaly (Balmino et al., 2012). b) Reconstructed crustal thickness (Laske et al., 2013). Magmatic ages with "N-" prefix are from Normade et al. (2007) and other ages are from Davies et al. (2017): FZD, Foum Zguid Dyke; HS, Hodh Sill; KI, Kakoulima Intrusion; MD, Messejana Dyke; and SD, Shelburne Dyke. Other abbreviations: AFR, Africa; NA, North America; and SA, South America. Click here or on Figure for enlargement.
CAMP magmatism occurred in extremely intense, relatively brief episodes around 201 Ma. However, the tectonic activity that led to Pangaea breakup in this region was much more prolonged, starting as far back as the Upper Carboniferous ~100 Ma before the CAMP erupted (Frizon De Lamotte et al., 2015).
CAMP magmas are clearly derived from different compositions of sub-lithospheric mantle, some with substantial subduction contamination, in different regions and across an extensive geographic area. The magmas are not all related to a single centre (Whalen et al., 2015). A preferred genesis model for the CAMP is thus the tectonic release of mantle melts that formed as a result of thermal insulation beneath the vast Pangaean supercontinent, possibly volumetrically enhanced by inherited fertile mantle compositions (Merle et al., 2013).
2. Middle Jurassic breakup between East and West Gondwana
Breakup of East and West Gondwana during Early Jurassic times marked the end of the Gondwana supercontinent (Phethean et al., 2016; Veevers, 2004). Along the central Gondwana rift, two oceanic basins formed–the West Somali Basin, extending from southern Somalia to northern Mozambique, and further south the Mozambique Basin, which is conjugate to the Riiser Larsen Sea/Lazarev Sea, Antarctica (Klimke & Franke, 2016) (Figure 3).
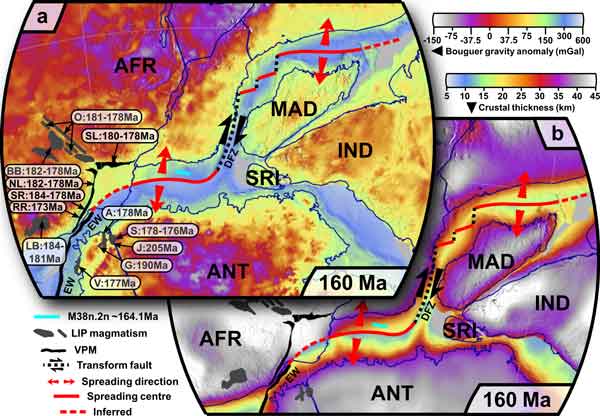
Figure 3: Breakup of East and West Gondwana at 160 Ma. a) Reconstructed Bouguer gravity anomaly (Balmino et al., 2012). b) Reconstructed crustal thickness (Laske et al., 2013). Magmatic ages are from Hastie et al. (2014) with the abbreviations: A, Alhmannryggen dyke swarm; BB, Botswana basalts; G, Group 1 dykes; J, Jutulrora dyke swarm; LB, Lesotho basalts; NL, Northern Lebombo dyke swarm; O, Okavango dyke swarm; RR, Rooi rand dyke swarm; S, Straumsvola dyke swarm; SL, Save-Limpopo dyke swarm; SR, Sabie River formation; and V, Vestfjella dyke swarm. Other abbreviations: AFR, Africa; ANT, Antarctica; DFZ, Davie Fracture Zone; EW, Explora Wedge; IND, India; MAD, Madagascar; and SRI, Sri Lanka. Click here or on Figure for enlargement.
Breakup followed a prolonged phase of episodic activity along the Karoo rift system and was closely contemporaneous with eruption of the Karoo-Ferrar flood basalts and formation of the Lebombo volcanic monocline in Mozambique. Understanding of the timing and kinematics of the Western Somali Basin, and to the south of this the Mozambique Basin and its conjugate the Riiser Larsen Sea/Lazarev Sea, Antarctica, is still incomplete. As a result, it is difficult to derive a final and conclusive model for the relationship between the Jurassic breakup of East and West Gondwana that includes formation of the Mwenezi triple junction and eruption of the Karroo-Ferrar LIP. However, it is clear that the triple junction structure was not the result of active extensional forces radiating from Mwenezi, and magmatism instead followed pre-existing lithospheric structures.
The massive magmatic extrusion that formed the Karoo-Ferrar LIP likely predates rifting, but breakup and formation of the oceanic basins did not initiate close to the triple junction. It is therefore more likely that the rift and subsequent breakup migrated towards the triple junction. Nevertheless, work is still needed to fully understand the relationship between the Jurassic breakup of East and West Gondwana and the formation of the Mwenezi triple junction and the Karroo-Ferrar LIP.
3. Early Cretaceous opening of the South Atlantic
In the Early Cretaceous, West Gondwana, a southern constituent of Pangaea, broke up to form South America and Africa with continuous spreading resulting in the sustained expansion of the South Atlantic Ocean (Lovecchio et al., 2018) (Figure 4). Continental extension may have begun in isolated centres in South America during the Late Triassic (at about 210 Ma) when almost all parts of south and west Gondwana were affected by magmatism. In addition to this Late Triassic to Early Jurassic rifting phase, there was an extensional phase lasting almost 40 Ma, from Valanginian to late Albian time (Early Cretaceous), that completed separation of Africa and South America.
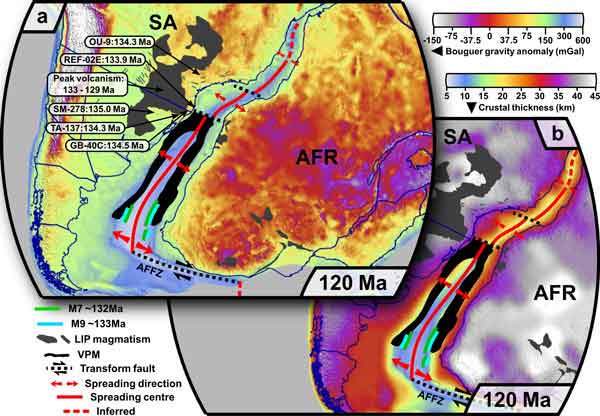
Figure 4: Breakup of the Southern Atlantic at 120 Ma. a) Reconstructed Bouguer gravity anomaly (Balmino et al., 2012). b) Reconstructed crustal thickness (Laske et al., 2013). Peak volcanism is from Hawkesworth et al. (2000) and other ages are from the Baksi (2018). AFR, Africa; SA, South America; and AFFZ, Agulhas-Falkland Fracture Zone. Click here or on Figure for enlargement.
The Paraná and Etendeka continental flood-basalt provinces in Brazil and Namibia, respectively, which are contemporaneous with breakup, are frequently attributed to an Early Cretaceous Tristan da Cunha mantle plume with the Walvis Ridge and Rio Grande Rise representing plume-tail magmatism. However, there are significant spatial and temporal mismatches between the proposed plume and these structures. Specifically, the South Atlantic opened by south-to-north propagation. This opening direction contradicts the hypothesis that rifting migrated away from the Paraná–Etendeka flood-basalt provinces (Franke, 2013). On the contrary, rifting migrated towards it. This is at odds with a model whereby continental breakup was triggered by an actively upwelling mantle plume that currently underlies the Tristan da Cunha region.
4. Mesozoic-Cenozoic formation of the North Atlantic
Prior to breakup, the proto-North Atlantic region comprised an assemblage of Archaean and Proterozoic terranes (Schiffer et al., 2019). Following prolonged regional rifting, propagation of the Central Atlantic into the proto-North Atlantic was achieved in the Early Aptian (Barnett-Moore et al., 2018) (Figure 5). By the Santonian (Late Cretaceous), breakup had reached the Charlie Gibbs Fracture Zone (CGFZ) and this was shortly followed by the opening of the NW Atlantic (Labrador Sea and Baffin Bay).
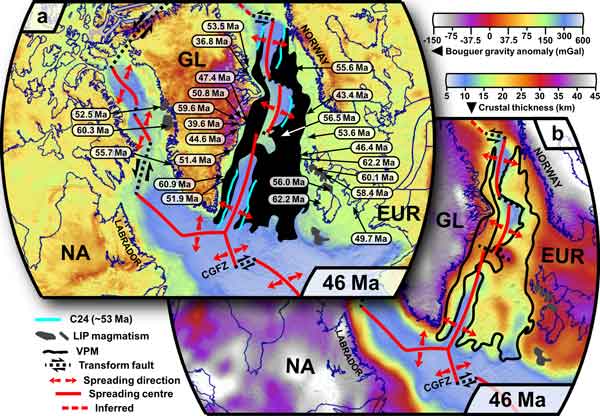
Figure 5: Breakup of the North Atlantic at 46 Ma. a) Reconstructed Bouguer gravity anomaly (Balmino et al., 2012). b) Reconstructed crustal thickness (Laske et al., 2013). A representative selection of magmatism ages are shown from the NAGTEC project (Wilkinson et al., 2017). NA, North America; GL, Greenland; EUR; Europe; and CGFZ, Charlie-Gibbs Fracture Zone. Click here or on Figure for enlargement.
During the Early Eocene, seafloor spreading began in the NE Atlantic, marking a major tectonic reorganisation (Gaina et al., 2017). A triple junction formed between the Labrador Sea, the NE Atlantic, and the southern North Atlantic, which was active until spreading ceased in the Labrador Sea in the earliest Oligocene. In the NE Atlantic, the Ægir Ridge may represent the southern tip of a southward-propagating Arctic rift system that migrated west to form the Kolbeinsey Ridge (Gernigon et al., 2019). Rifting and breakup of the northern North Atlantic was accompanied by widespread magmatism (Clarke & Beutel, 2019; Hole & Natland, 2019).
Although magmatism during and after breakup formed the North Atlantic Igneous Province (NAIP), breakup of the North Atlantic was a complex, poly-phase process, accompanied by numerous compositionally highly variable magmatic events (Foulger et al., 2019). These cannot be explained by a singular mechanism. Thus, we suggest that continental breakup and associated magmatism across the North Atlantic region was driven by lithospheric processes associated with plate tectonics.
Concluding remarks
Our review found that throughout the dispersal of Pangaea, large-volume volcanism including LIP eruptions, typically occurred distal from the locus of rift initiation and initial oceanic crust accretion. There is no location where extension propagates away from a newly formed LIP. Instead, LIPs coincide with major lithosphere-scale shear structures, aborted rifts and splinters of continental crust rifted far out into the oceanic domain. This highlights a spatial-temporal mismatch between the locations of LIPs and the initiation points of Pangaea disintegration.
None of the regions we reviewed fit comfortably a plume-driven breakup model that predicts pre-breakup magmatism, plume tail eruptions producing ocean island chains, and rifting radiating from the point of proposed plume impact. In contrast, most show multiple characteristics that are not fully compatible with this model including a reverse chronology of uplift, magmatism and rifting, and rifting propagating towards LIPs instead of away from them. The idealised, generic, plume-impingement model thus has difficulties explaining the dispersal of Pangaea and associated magmatism.
Rifting and breakup driven primarily by far-field extensional forces, with magmatism occurring as a consequence and under strong lithospheric control is much more consistent with observations that are common throughout the regions we reviewed. These observations suggest that a fundamental reappraisal of the causes and consequences of breakup-related LIP formation is in order.
References
-
Baksi, A.K., 2018, Paraná flood basalt volcanism primarily limited to ~ 1 Myr beginning at 135 Ma: New 40Ar/39Ar ages for rocks from Rio Grande do Sul, and critical evaluation of published radiometric data: Journal of Volcanology and Geothermal Research, doi:10.1016/j.jvolgeores.2017.02.016.
-
Balmino, G., Briais, A., Kuhn, M., Peyrefitte, A., Vales, N., Biancale, R., Gabalda, G., Reinquin, F., and Sarrailh, M., 2012, World Gravity Map: Commission for the geological map of the world, v. BGI-CGMW-C.
-
Barnett-Moore, N., Müller, R.D., Williams, S., Skogseid, J., and Seton, M., 2018, A reconstruction of the North Atlantic since the earliest Jurassic: Basin Research, v. 30, p. 160–185, doi:10.1111/bre.12214.
-
Biari, Y., Klingelhoefer, F., Sahabi, M., Funck, T., Benabdellouahed, M., Schnabel, M., Reichert, C., Gutscher, M.A., Bronner, A., and Austin, J.A., 2017, Opening of the central Atlantic Ocean: Implications for geometric rifting and asymmetric initial seafloor spreading after continental breakup: Tectonics, p. 1–22, doi:10.1002/2017TC004596.
-
-
Davies, J.H.F.L., Marzoli, A., Bertrand, H., Youbi, N., Ernesto, M., and Schaltegger, U., 2017, End-Triassic mass extinction started by intrusive CAMP activity: Nature Communications, v. 8, p. 1–8, doi:10.1038/ncomms15596.
-
Foulger, G.R., T. Doré, C.H. Emeleus, D. Franke, L. Geoffroy, L. Gernigon, R. Hey, R.E. Holdsworth, M. Hole, Á. Höskuldsson, B. Julian, N. Kusznir, F. Martinez, J.H. Natland, A.L. Peace, K. Petersen, C. Schiffer, R. Stephenson, and M. Stoker, 2019. The Iceland Microcontinent and a continental Greenland-Iceland-Faroe Ridge, Earth-Science Reviews, in press.
-
Franke, D., 2013, Rifting, lithosphere breakup and volcanism: Comparison of magma-poor and volcanic rifted margins: Marine and Petroleum Geology, v. 43, p. 63–87, doi:10.1016/j.marpetgeo.2012.11.003.
-
Frizon De Lamotte, D., Fourdan, B., Leleu, S., Leparmentier, F., and De Clarens, P., 2015, Style of rifting and the stages of Pangea breakup: Tectonics, v. 34, p. 1009–1029, doi:10.1002/2014TC003760.
-
Gaina, C., Nasuti, A., Kimbell, G.S., and Blischke, A., 2017, Break-up and seafloor spreading domains in the NE Atlantic: Geological Society of London Special Publications, v. 447, p. 393–417, doi:10.1144/SP447.12.
-
Gernigon, L., D. Franke, L. Geoffroy, C. Schiffer, G.R. Foulger, and M. Stoker, 2019. Crustal fragmentation, magmatism, and the diachronous opening of the Norwegian-Greenland Sea, Earth-Science Reviews, in press.
-
Hansen, J., Jerram, D.A., McCaffrey, K., and Passey, S.R., 2009, The onset of the North Atlantic Igneous Province in a rifting perspective: Geological Magazine, v. 146, p. 309, doi:10.1017/S0016756809006347.
-
Hastie, W.W., Watkeys, M.K., and Aubourg, C., 2014, Magma flow in dyke swarms of the Karoo LIP: Implications for the mantle plume hypothesis: Gondwana Research, v. 25, p. 736–755, doi:10.1016/j.gr.2013.08.010.
-
Hawkesworth, C.J., Gallagher, K., Kirstein, L., Mantovani, M.S.M., Peate, D.W., and Turner, S.P., 2000, Tectonic controls on magmatism associated with continental break-up: An example from the Paraná-Etendeka Province: Earth and Planetary Science Letters, v. 179, p. 335–349, doi:10.1016/S0012-821X(00)00114-X.
-
-
Klimke, J., and Franke, D., 2016, Gondwana breakup: no evidence for a Davie Fracture Zone offshore northern Mozambique, Tanzania and Kenya: Terra Nova, v. 28, p. 233–244, doi:10.1111/ter.12214.
-
Laske, G., and Masters, G., 2013, Update on CRUST1. 0 - A 1-degree global model of Earth’s crust: EGU general assembly 2013, v. 15, p. 2658, http://meetingorganizer.copernicus.org/EGU2013/EGU2013-2658.pdf.
-
Lovecchio, J.P., Rohais, S., Joseph, P., Bolatti, N.D., Kress, P.R., Gerster, R., and Ramos, V.A., 2018, Multistage rifting evolution of the Colorado basin (offshore Argentina): Evidence for extensional settings prior to the South Atlantic opening: Terra Nova, v. 30, p. 359–368.
-
Marzoli, A., Renne, P.R., Piccirillo, E.M., Ernesto, M., Bellieni, G., and De Min, A., 1999, Extensive 200-million year old continental flood basalts of the Central Atlantic Magmatic Province: Science, v. 284, p. 616–618.
-
Merle, R. et al., 2013, Sr, Nd, Pb and Os Isotope Systematics of CAMP Tholeiites from Eastern North America (ENA): Evidence of a Subduction-enriched Mantle Source: Journal of Petrology, v. 55, p. 133–180.
-
Nomade, S., Knight, K.B., Beutel, E., Renne, P.R., Verati, C., Féraud, G., Marzoli, A., Youbi, N., and Bertrand, H., 2007, Chronology of the Central Atlantic Magmatic Province: Implications for the Central Atlantic rifting processes and the Triassic-Jurassic biotic crisis: Palaeogeog., Palaeoclim., Palaeoecol., v. 244, p. 326–344, doi:10.1016/j.palaeo.2006.06.034.
-
Pastor-Galán, D., Nance, R.D., Murphy, J.B., and Spencer, C.J., 2018, Supercontinents: myths, mysteries, and milestones: Geological Society of London, Special Publication,.
-
Peace, A.L., Phethean, J.J.J., Franke, D., Foulger, G.R., Schiffer, C., Welford, J.K., McHone, G., Rocchi, S., Schnabel, M., and Doré, A.G., 2019, A review of Pangaea dispersal and Large Igneous Provinces – In search of a causative mechanism: Earth-Science Reviews, v. 196, doi:10.1016/j.earscirev.2019.05.009.
-
Phethean, J.J.J., Kalnins, L.M., van Hunen, J., Biffi, P.G., Davies, R.J., and McCaffrey, K.J.W., 2016, Madagascar’s escape from Africa: A high-resolution plate reconstruction for the Western Somali Basin and implications for supercontinent dispersal: Geochemistry, Geophysics, Geosystems, v. 17, p. 5036–5055, doi:10.1002/2016GC006624.
-
Schiffer, C., Doré, A.G., Foulger, G.R., Franke, D., Geoffroy, L., Gernigon, L., Holdsworth, B., Kusznir, N., Lundin, E., and McCaffrey, K., 2019, Structural inheritance in the North Atlantic: Earth-Science Reviews, p. 102975.
-
Scotese, C.R., 2016, PALEOMAP PaleoAtlas for GPlates and the PaleoData Plotter Program, PALEOMAP Project:, doi:10.1130/abs/2016NC-275387.
-
Stampfli, G.M., Hochard, C., Vérard, C., Wilhem, C., and vonRaumer, J., 2013, The formation of Pangea: Tectonophysics, v. 593, p. 1–19, doi:10.1016/j.tecto.2013.02.037.
-
Veevers, J.J., 2004, Gondwanaland from 650–500 Ma assembly through 320 Ma merger in Pangea to 185–100 Ma breakup: supercontinental tectonics via stratigraphy and radiometric dating: Earth-Science Reviews, v. 68, p. 1–132, doi:https://doi.org/10.1016/j.earscirev.2004.05.002.
-
Whalen, L., Gazel, E., Vidito, C., Puffer, J., Bizimis, M., Henika, W., and Caddick, M.J., 2015, Supercontinental inheritance and its influence on supercontinental breakup: The Central Atlantic Magmatic Province and the breakup of Pangea: Geochemistry, Geophysics, Geosystems, v. 16, p. 3532–3554, doi:10.1002/2015GC005885.
-
Wilkinson, C.M., Ganerød, M., Hendriks, B.W.H., and Eide, E.A., 2017, Compilation and appraisal of geochronological data from the North Atlantic Igneous Province (NAIP): Geological Society Special Publication, v. 447, p. 69–103, doi:10.1144/SP447.10.
last updated 20th
December, 2019 |