 |
 |
Stress
Induced Hotspot Seamount Formation at Ridge-Transform Intersections
|
|
|
Contrary to our current understanding of ridge-hotspot
interactions, several papers have noted small seamounts with hotspot
geochemical signatures located at or near ridge-transform-intersections
(RTIs) rather than at the center of ridge segments (Graham et al.,
1996; Hekinian et al., 1999; Johnson et al., 2000;
Klingelhofer et al., 2001). Accounting for the presence
of hotspot seamounts at ridge-transform intersections requires that
1) magma must be diverted or funneled to the ridge-transform intersection
rather than the ridge center, and 2) this process must be governed by
the lithosphere, as magma movement at the ridge appears to be governed
by the lithosphere.
The existence of seamounts with hotspot geochemical
signatures (hereafter simply called hotspots with no implied inference
to their origin) in the center of ridges is relatively well understood.
In the case of a plume origin for hotspots, ridges are believed to focus
plumes: a) because hotspots are entrained in the mantle upwelling that
feeds the ridges, b) because they are weak areas within the crust, and
c) because there exists a physical and thermal gradient that effectively
funnels the material to the ridge like a sink to a drain (Figure1) (Kincaid
et al., 1996; Kincaid et al., 1995; Sleep, 1990).
Once at the ridge, hotspots add magma to the central area of upwelling,
i.e. the center of the ridge segment (Figure 1). In the case of a heterogeneous
mantle origin for hotspots, ridges are believed to intersect areas of
fertile mantle, which results in rapid decompression melting and seamount
formation (Anderson, 1992). Neither theory offers a clear
explanation for the presence of hotpots at ridge-transform-intersections.
|
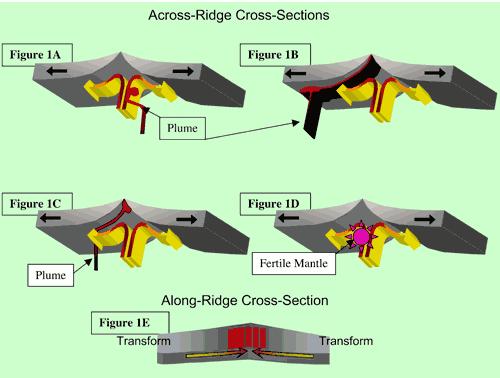
Figure 1: (A-D): Possible Ridge-Hotspot Interactions
(e.g. Kincaid et al., 1996, 1995; Sleep, 1990)
A: Plume is entrained in shallow upwelling beneath ridge.
B: Cartoon of a hotspot supplying material to a ridge via a conduit flowing
up the topography of the ridge and overcoming the mantle flow away from
the ridge.
C: Cartoon of a hotspot entrained to a ridge by flowing along the base
of the lithosphere, also overcoming the mantle flow away from the ridge.
D: Cartoon of fertile mantle body intersecting upwelling magma.
E: Along ridge cross-section showing flow of melt material to the physiographic
high point near the center of the ridge. |
Based on the above physical constraints on magma movement at the ridge,
excess magmatism at the ridge-transform-intersection can only occur
if the physiographic and thermal gradients that funnel magma to the
ridge center are either overcome or a local upwelling is developed.
Changing the stress state of the lithosphere and creating areas of local
decompression melting due to large-scale extension may cause local upwellings
and/or change the physiographic gradient along the ridge. Previous
work (Beutel & Okal, in press) suggests that large extensional
stresses are concentrated at ridge-transform intersections during times
of impeded slip along transforms. To test this, and to examine
the three-dimensional stresses associated with impeded transform slip,
three-dimensional finite element models of a series of ridge segments
were constructed.
The elastic finite element program FElt by Gobat & Atkinson
(1996) was used to construct a box model of 3 ridge segments 1200-1400
km long separated by transforms 600-800 km long. The ridges are
modeled as weak areas in a relatively strong 100 km thick lithosphere
that is underlain by a 100 km weak low-velocity zone and then 400 km
of mantle. An exponentially decreasing, gravitationally driven,
ridge-push force based on lithospheric age was applied from the each
ridge segment to the edge of the model. A variety of scenarios
involving different transform strengths (i.e. ease of slip) and force
ratios were run to determine what effect changing transform strength
had on the stress field at the ridge-transform intersection. In
all cases, increasing the resistance to slip along the transform resulted
in increased extensional stress at the RTI in the lithosphere, and a
change in the stress-state in the mantle (Figure 2).
|
Mapview of X-Y stresses in the lithosphere
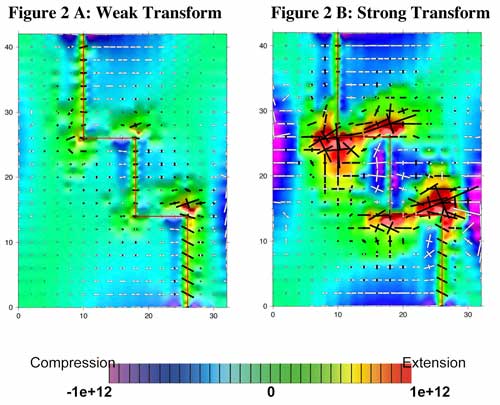
Figure 2: Mapview results, 33 km Depth: Results
of ridge-push forces exerted on a 3D box with 3 ridges and 2 transforms.
The model is fixed at the base of the box, 8 units (~800 km) below the
surface. The background colors indicate the type and intensity of the
maximum stress as indicated on the scale. The bars are maximum and minimum
stress vectors, white indicates compression and black indicates extension.
White arrows indicate zones of extension at ridge-transform-intersections.
A: Transforms in this model are the 3 orders of magnitude weaker than
the surrounding lithosphere. Note the concentration of extensional stress
at the ridge-transform intersection.
B: Transforms in this model are the same strength as the surrounding
lithosphere. Note the very large concentrations of extensional stress
at the ridge-transform intersections compared to the model with a weak
transform.
|
While ridge propagation may result from the increased extensional stress
at the ridge-transform intersection it is also feasible to develop a
number of scenarios whereby excessive extensional stresses at an RTI
would result in the formation of a hotspot volcano:
-
as mentioned by Henkinian et al. (1999),
is that the formation of hotspot volcanoes occurs during ridge propagation.
In this scenario, hotspot volcanoes would be formed due to excess
magmatism associated with the propagation of the crack tip and accompanying
extensional stresses over fertile mantle bodies.
-
the large extensional stresses at the ridge-transform
intersection may cause normal faulting and lithospheric thinning in
an orientation incompatible with ridge propagation. Lithospheric thinning
and large extensional stresses may result in decompression melting
of the upper mantle and a local upwelling.
In the case of a heterogeneous mantle, decompression
melting of fertile mantle would result in an excess of melt with a hotspot
signature. Decompression of the mantle and the formation of a melt
would also a change the thermal and physical gradient at the ridge.
Crust at the transforms tends to be thinner than at the center of the
ridge and the presence of melt would increase the positive buoyancy of
the crust. This change in the physical, and possibly thermal gradient,
could draw plumes from the center of the ridge to the ridge-transform-intersection
(Figure 3). The formation of the hotspot volcano may then cause
the transform to become unlocked, thereby preventing ridge-propagation. |
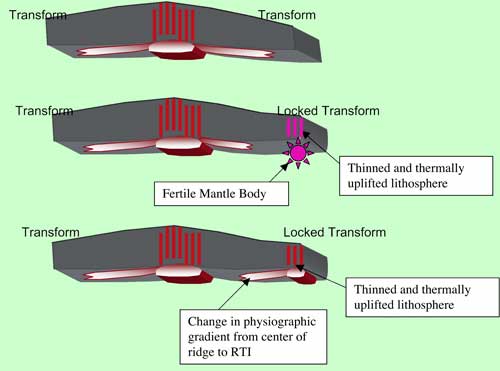
Figure 3: Along ridge cross-section. Upper
cartoon shows steady a state-ridge with a weak transform and
the attendant melt directed towards the ridge center. Middle
cartoon shows thinned lithosphere and extensional stress, resulting
from a locked transform, over a fertile magma body which leads to the
formation of a hotspot volcano. Bottom cartoon
shows a change in the physiographic gradient resulting from decompression
melting at a ridge-transform intersection due to impeded slip along
the transform.
|
Conclusions
The presence of seamounts with hotspot geochemical signatures at ridge-transform-intersections
may be caused by extensional stress concentrations. Extensional
stress is concentrated at ridge-transform intersections when slip along
the transform in impeded. Therefore hotspot locations may be guided
by lithospheric processes and stress-states. If a changing lithospheric
stress-state can affect the location of hotspot seamounts at the ridge,
then it is important consider the effect of lithospheric stress on all
hotspots.
|
-
Anderson, D. L; Tanimoto, Toshiro; Zhang, Yu-shen,
1992, Plate tectonics and hotspots; the third dimension: Science,
256, 1645-1651.
-
Graham, D W; Castillo, P R; Lupton, J E; Batiza,
R, 1996, Correlated He and Sr isotope ratios in South Atlantic near-ridge
seamounts and implications for mantle dynamics: Earth Planet.
Sci. Lett., 144, 491-503.
-
Gobat, J. I. and Atkinson, D. C., 1996, FElt:
User's Guide and Reference Manual: San Diego, University of California,
San Diego.
-
Hekinian, R.; Stoffers, P.; Ackermand, D.; Revillon,
S.; Maia, M.; Bohn, 1999, Marcel Ridge-hotspot interaction; the Pacific-Antarctic
Ridge and the Foundation seamounts: Marine Geology, 160,
pp.199-223.
-
Johnson, K T M; Graham, D W; Rubin, K H; Nicolaysen,
K; Scheirer, D S; Forsyth, D W; Baker, E T; Douglas-Priebe, L M, 2000,
Boomerang Seamount; the active expression of the Amsterdam-St. Paul
Hotspot, Southeast Indian Ridge: Earth Planet. Sci. Lett.,
183, 245-259.
-
Kincaid, C; Schilling, J G; Gable, C, 1996, The
dynamics of off-axis plume-ridge interaction in the uppermost mantle:
Earth Planet. Sci. Lett., 137, 29-43.
-
Kincaid, C; Ito; G; Gable, C., 1995, Laboratory
investigation of the interaction of off-axis mantle plumes and spreading
centres: Nature, 376, 758-761.
-
Klingelhofer, F.; Minshull, T.A.; Blackman, D.K.;
Harben, P.; Childers V., 2001, Crustal structure of Ascension Island
from wide-angle seismic data: implications for the formation of near-ridge
volcanic islands: Earth Planet. Sci. Lett., 190,
41-56.
-
Sleep, Norman H., 1990, Hotspots and mantle plumes;
some phenomenology: J. Geophys. Res., 95,
6715-6736.
|
|
|