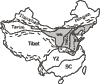 |
Recycling
deep cratonic lithosphere and generation of intraplate
magmatism in the North China Craton |
Shan Gaoa,b, Roberta
L. Rudnickc,
Wen-Liang Xud, Hong-Lin Yuanb,
Yong-Sheng Liua, Richard J. Walkerc,
Igor S. Puchtelc, Xiaomin Liub,
Hua Huanga, Xiao-Rui Wanga &
Jie Yanga
aState Key Laboratory of
Geological Processes and Mineral Resources, China University
of Geosciences, Wuhan 430074, China
bState
Key Laboratory of Continental Dynamics, Department
of Geology, Northwest University, Xi’an 710069,
China
cGeochemistry Laboratory,
Department of Geology, University of Maryland College
Park, MD 20742, USA
dSchool of Earth Sciences,
Jilin University, Changchun 130061, China
Corresponding
author: sgao@263.net (Shan
Gao).
Click here to
download a PDF version of this webpage
This webpage is a summary of the paper
Gao, S., Rudnick, R.L., Xu, W.L.,Yuan, H.L., Liu, Y.S., Walker,
R.J., Puchtel, I.S., Liu, X.M., Huang, H., Wang, X.R. and Yang,
J., 2008. Recycling deep cratonic lithosphere and
generation of intraplate magmatism in the North
China craton. Earth Planet. Sci. Lett. (in
press). |
Abstract
Early Cretaceous alkaline
picrites and high-magnesium basalts from the North
China craton provide evidence for recycling of continental
lithosphere by density foundering. Both the picrites
and basalts contain xenocrystic olivines with high
Fo92-93 and low CaO (<0.10%), consistent
with the lavas’ derivation
from, or interaction with Archean mantle lithosphere.
Most importantly, both the picritic
and basaltic lavas contain unusual, reversely zoned
clinopyroxene phenocrysts whose cores have low MgO,
high Na2O
(up to 2.4 wt.%, or 17.3 mol%Jd), and frequently
contain ilmenite exsolution lamellae, consistent
with their crystallization from an eclogite-derived
melt (tonalite or trondhjemite). In contrast,
the clinopyroxene exteriors have low Na2O
(<0.92 wt.%, or <6.5
mol% Jd) and are lamellae-free, suggesting crystallization
from a mantle-derived melt (picrite or basalt). Both
the cores and exteriors have high Al2O3 contents
(up to 6.9 wt.%). These features reflect crystallization
of the cpx from an aluminous melt at mantle depths,
with the cores forming at a significantly greater
depth (> 2.5 GPa) than the surrounding
cpx (> 1.5
GPa). Calculated primary melt compositions further
constrain the magmas’ formation at 3-4 GPa,
in the presence of garnet. The unusually low CaO,
high Ni/MgO and low 100Mn/Fe of primary melts indicate
derivation of both the picritic and basaltic lavas
from pyroxenite sources containing limited or no
olivine. High Sr/Y, LaN/YbN and Th/U and low Lu/Hf,
together with radiogenic initial 87Sr/86Sr
and 187Os/188Os ratios and
negative εNd values implicate contributions from
melts derived from foundered eclogitic lower continental
crust. Modelling
suggests that the basalt source region contained
a variable proportion (30-40%) of eclogite-derived
component whereas the source of the picrites, on
average, likely contained a generally higher proportion
(60-70%) of a different eclogite-derived component.
Collectively, these results
suggest that both the basaltic and picritic lavas
originated by partial melting of Archean lithospheric
mantle that was variably hybridised by melts derived
from foundered lower crustal eclogite. Together with
previous studies, these findings provide new evidence
that thinning of the North China craton was caused
by the removal of the lower lithosphere (mantle and
lower crust). Recycling and melting of eclogitic
lower crust may contribute more to mantle heterogeneity
than has previously been recognized.
Introduction
Recycling of eclogite
of lower continental crust origins, together with the
underlying lithospheric mantle, has been proposed to
play a role in plume magmatism, crustal evolution and
formation of chemical heterogeneities within the mantle
(Arndt & Goldstein, 1989; Kay
& Kay, 1991; Jull
& Kelemen, 2001; Escrig
et al., 2004; Gao et al.,
2004; Elkins-Tanton, 2005; Lustrino,
2005; Anderson,
2006). Eclogites have lower melting temperatures than
mantle peridotites (Yaxley & Green, 1998; Yaxley,
2000; Rapp et al., 1999; Kogiso
et al., 2003; Sobolev
et al., 2005, 2007), and as foundered, silica-saturated
eclogites heat up, they will produce silicic melts
(tonalite to trondhjemite) that may react extensively
with overlying mantle peridotite. Such
reaction may produce an olivine-free pyroxenite, which,
if subsequently melted, will generate basaltic melt
(Kogiso et al., 2003; Sobolev
et al., 2005, 2007; Herzberg,
2006).
Although recycling of eclogite in
subducted oceanic lithosphere is a direct consequence
of plate tectonics and its consequences for mantle
composition have been extensively studied (e.g.,
Hawaii) (Hofmann & White, 1982; Sobolev
et al., 2005, 2007;
Herzberg, 2006), recycling of eclogite formed
in deep continental lithosphere is more controversial
and only a few studies have considered its effect on
the composition of mantle derived magmas (McKenzie
& O'Nions, 1983;
Arndt & Goldstein, 1989; Escrig
et al., 2004; Elkins-Tanton,
2005; Lustrino, 2005; Anderson, 2006).
Here we present petrographic and geochemical evidence
that Mesozoic basalts and picrites from the North China
craton derive from mantle that was modified by interaction
with melts from foundered eclogite.
The North China Craton
The North China
craton (NCC; Figure 1) is one of the world’s
oldest Archean cratons, preserving crustal remnants
as old as 3800 Ma (Liu et al., 1992). The
NCC is also one of the world’s most unusual
cratons, as the eastern block was reactivated in
the Mesozoic. This portion of the craton had
a cold and thick lithosphere, typical of other Archean
cratons, at least through the Ordovician, when kimberlites
erupted that carried diamonds and refractory garnet
peridotites (Menzies et
al., 1993; Griffin
et al., 1998), the latter of which have Archean
Os model ages (Gao et al., 2002; Wu
et al., 2006; Zhang
et al., 2008).
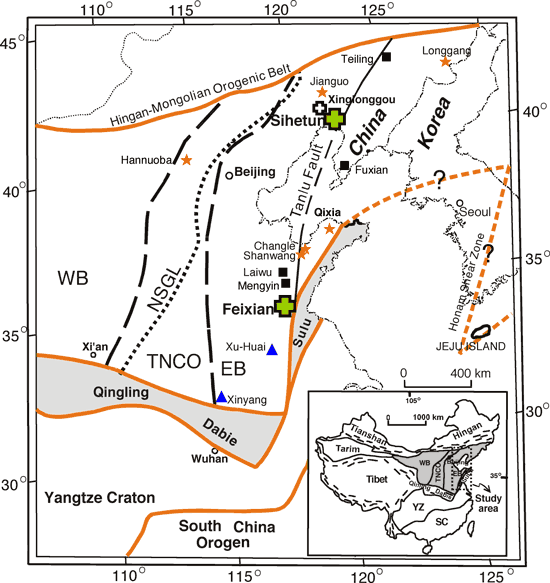
Figure 1: Geologic sketch map of the
North China craton (shaded on inset). The two suites
of Early Cretaceous lavas under investigation are shown
as large filled crosses. Click here for fuller figure
caption.
Reactivation of the craton began
in the Early Mesozoic, with uplift and the onset
of magmatism, followed by basin development. The
magmatism peaked volumetrically in the Late Cretaceous
(120-132 Ma; Wu et al., 2005). This
early, compositionally diverse magmatism was followed
by Cenozoic intraplate basaltic volcanism. Cenozoic
basalts in the Eastern Block carry mantle xenoliths
that equilibrated to a high geotherm (Xu, 2001; Zheng
et al., 2006), have a relatively fertile bulk composition
(Menzies et al., 1993; Griffin et
al., 1998; Rudnick
et al., 2004) and Os isotopic compositions similar
to the modern convective mantle (Gao
et al.,
2002; Wu et al., 2003, 2006).
The above observations
have been used to suggest that ancient, cratonic mantle
lithosphere, similar to that present beneath the Kaapvaal,
Siberian and other Archean cratons, was removed from
the base of the Eastern Block of the NCC during the
Mesozoic, and was replaced by younger, less refractory
lithospheric mantle. Whether the replacement was caused
by foundering, stretching or thermal/chemical erosion
of the deep lithosphere due to upwelling asthenosphere
is a matter of great debate (Xu, 2001; Gao
et al.,
2004; Wu et al., 2005; Menzies et
al., 2007; Zhang
et al., 2007; Huang et al., 2007).
Two suites of Early Cretaceous
mafic magmas are investigated here: the Sihetun
high-Mg basalts (124–125 Ma),
which erupted in western Liaoning Province, and the Feixian alkaline picrites
(119 Ma), which erupted in western Shandong Province (Figure 1).
Evidence for recycling
deep cratonic lithosphere and generation of intraplate
magmatism
Both the picrites and basalts contain
xenocrystic olivines with high Fo92-93 and
low CaO (<0.10%), consistent
with the lavas’ derivation from, or interaction
with, Archean mantle lithosphere (Figure 2). Most importantly,
both the picritic and basaltic lavas contain unusual,
reversely zoned clinopyroxene phenocrysts whose cores
have low MgO, high Na2O (up to 2.4 wt.%,
or 17.3 mol%Jd; Figure 3), and frequently contain
ilmenite exsolution lamellae, consistent with their
crystallization from an eclogite-derived melt (tonalite
or trondhjemite). In
contrast, the clinopyroxene exteriors have low Na2O
(<0.92 wt.%, or <6.5 mol% Jd) (Figure 3) and
are lamellae-free, suggesting crystallization from
a mantle-derived melt (picrite or basalt). Both the
cores and exteriors have high Al2O3 contents
(up to 6.9 wt.%). These features reflect crystallization
of the cpx from an aluminous melt at mantle depths,
with the cores forming at a significantly greater depth
(> 2.5 GPa) than the
surrounding cpx (> 1.5 GPa). Calculated primary
melt compositions further constrain the magmas’ formation
at 3-4 GPa, in the presence of garnet (Figure 4a).
The unusually low CaO (Figure 4b), high Ni/MgO and
low 100Mn/Fe (Figure
4c) of primary melts indicate derivation of both the
picritic and basaltic lavas from pyroxenite sources
containing limited or no olivine. High Sr/Y, LaN/YbN
and Th/U and low Lu/Hf, together with radiogenic initial
87Sr/86Sr and 187Os/188Os
ratios and negative εNd values
implicate contributions from melts derived from foundered
eclogitic lower continental crust. Modelling
suggests that the basalt source region contained a
variable proportion (30-40%) of eclogite-derived component
whereas the source of the picrites, on average, likely
contained a generally higher proportion (60-70%) of
a different eclogite-derived component (Figure 5).
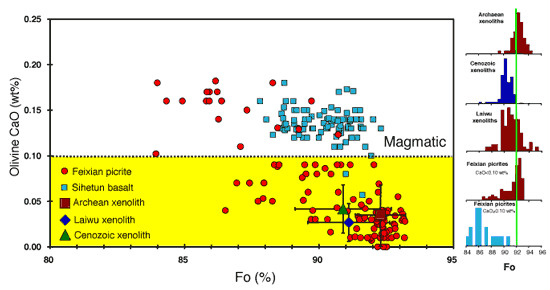
Figure 2: Left: Fo (forsterite
= 100Mg/(Mg+Fe), where Mg and Fe represent molar
proportions) versus wt.% CaO plot of olivine cores
from Early Cretaceous Feixian alkaline picrites and
Sihetun high-Mg basalts. Right: Fo histograms show
the systematic compositional
differences in olivines from different sources. Olivines
from the Feixian picrites, with CaO > 0.10%,
have Fo < 92, consistent with a magmatic origin,
whereas those with CaO < 0.10% have Fo >92, consistent
with a xenocrystic origin. Click here or
on Figure for enlargement. Click here for
fuller figure caption.
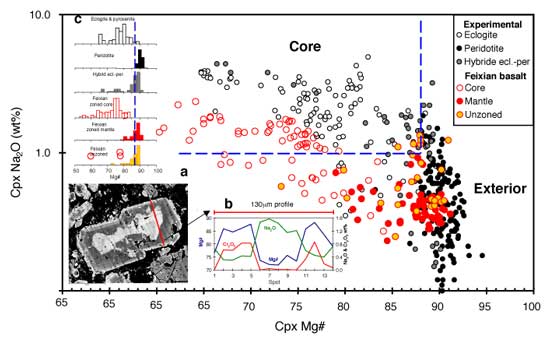
Figure 3: Core-exterior compositions
of reversely zoned clinopyroxene phenocrysts from the
Feixian alkaline picrites. (a) backscattered electron
image (BSE) and (b) compositional profile of a euhedral
clinopyroxene phenocryst along [010] plane from sample
SFX19. The dark areas are Mg-rich and the light areas
are Fe-rich. The main Mg# versus Na2O plot
and (c) Mg# histogram compare experimental clinopyroxenes
in equilibrium with melts derived from eclogite (including
garnet pyroxenite), peridotite and hybrid eclogite-peridotite.
Click here or
on Figure for enlargement. Click here for
fuller figure caption.
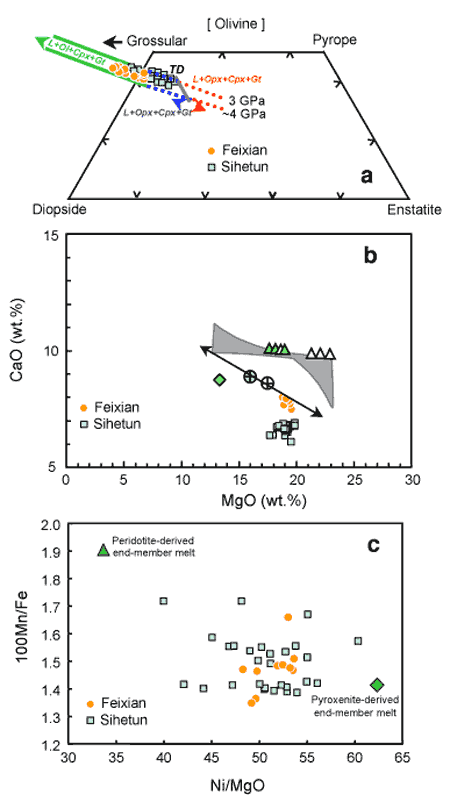
Figure 4: Compositions
of primary melts calculated for the Feixian alkaline
picrites and Sihetun high-Mg basalts. (a) Mole% projection
from or towards olivine into part of the pyroxene-garnet
plane compared with cotectics at 3 and 4 GPa (Herzberg,
2006). Thick line labelled “TD” is the
thermal divide between olivine-rich and SiO2-rich
sides of the composition space. (b) MgO versus CaO.
Filled and open triangles indicate primary melts and
solidus melts from peridotites (Herzberg,
2006; Sobolev
et al., 2007), while filled diamond represents
primary melt from pyroxenite (Sobolev et al.,
2007). Shaded area denotes accumulated fractional melt
compositions for a pressure range from 3 to 7 GPa (Herzberg,
2006). Filled and open circles with a cross indicate
high- and low-SiO2 Hawaiian parental magmas
(Herzberg,
2006). Arrows display the effects of olivine addition
(right pointing) and subtraction (left pointing; Herzberg,
2006). (c) Ni/MgO versus 100Mn/Fe ratios of primary melts
compared to experimentally produced peridotite and
pyroxenite-derived end-member melts
[supplementary Table S2 of Sobolev
et al. (2007)]. Click here for
fuller figure caption.
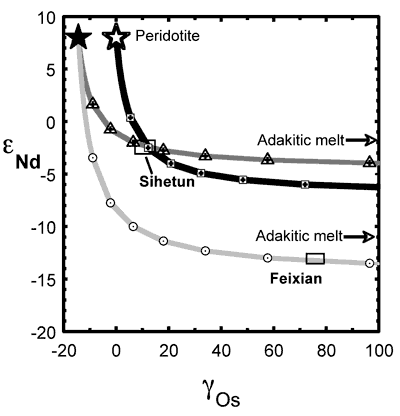
Figure 5: γOs
versus εNd mixing diagram for silicic melt-peridotite
mixtures as discussed in the text. Starting peridotite
compositions are shown as stars. Solid star reflects
ancient NCC peridotite. Open star is peridotite with
chondritic Os isotopes and the same concentrations
and Nd isotopes as ancient peridotite. Starting
adakitic melt compositions for the models are beyond
the scale of the figure. Triangles, squares
and circles show increments of 10% mixing of melt into
peridotite. Boxes show estimated compositions of Sihetun
basalt and Feixian picrite sources. Click here for
fuller figure caption.
Collectively,
these results suggest that both the basaltic and picritic
lavas originated by partial melting of Archean lithospheric
mantle that was variably hybridised by melts derived
from foundered lower crustal eclogite. Together with
previous studies, these findings provide new evidence
that thinning of the North China craton was caused
by the removal of the lower lithosphere (mantle and
lower crust). They further suggest that recycling and
melting of eclogitic lower crust may contribute more
to mantle heterogeneity than has previously been recognized.
Acknowledgments
We thank Claude Herzberg for calculation
of and constructive discussion on the primary
melt compositions and contributing Figure 4a. We thank
H.Y. He, G.M. Shu, X.C. Wang, and H. Zhang for help
in Ar-Ar dating, electron microprobe, chemical and
isotopic analysis, and field work.
References
-
Anderson, D.A., 2006. Speculations
on the nature and cause of mantle heterogeneity.
Tectonophysics 146, 7–22.
-
Arndt, N.T., Goldstein, S.L., 1989.
An open boundary between lower continental crust
and mantle: its role in crust formation and crustal
recycling. Tectonophysics 161, 201-212.
-
Chen, L.H.,
Zhou, X.H., 2006. Subduction-related metasomatism
in the thinning lithosphere: Evidence from a composite
dunite-orthopyroxenite xenolith entrained in Mesozoic
Laiwu high-Mg diorite, North China Craton. Geochem.
Geophys. Geosys. 6, Q06008, doi:10.1029/2005GC000938.
-
Elkins-Tanton,
L.T., 2005. Continental magmatism caused by lithospheric
delamination. In: Foulger, G.R. Natland, J.H.,
Presnall, D.C., Anderson, D.L. (Eds.), Plates,
Plumes, and Paradigms. Geol. Soc. Am. Spec. Pap.,
vol. 388, pp. 449–462.
-
Escrig, S. Capmas,
F., Dupré, B., Allégre,
C.J., 2004. Osmium isotopic constraints on the
nature of the DUPAL anomaly from Indian mid-ocean-ridge
basalts. Nature 431, 59–63.
-
Gao, S., Rudnick,
R.L., Carlson, R.W., McDonough, W.F., Liu, Y.S.
2002. Re-Os evidence for replacement of ancient
mantle lithosphere beneath the North China Craton.
Earth Planet. Sci. Lett. 198, 307–322.
-
Gao,
S., Rudnick, R.L., Yuan, H.-L., Liu, X.-M., Liu,
Y.-S., Xu, W.-L., Ling, W.-L., Ayers, J., Wang,
X.-C., Wang, Q.-H., 2004. Recycling lower continental
crust in the North China craton. Nature 432, 892–897.
-
Gao, S., Rudnick, R.L., Xu,
W.L.,Yuan, H.L., Liu, Y.S., Walker, R.J., Puchtel,
I.S., Liu, X.M., Huang, H., Wang, X.R. and Yang,
J., 2008. Recycling deep cratonic lithosphere and
generation of intraplate magmatism in the North
China craton.Earth Planet. Sci. Lett. (in press).
-
Griffin, W.L., Zhang, A.D.,
O'Reilly, S.Y., Ryan, C.G., 1998. Phanerozoic evolution
of the lithosphere beneath the Sino-Korean Craton.
In: Flower, M., Chung, S.-L., Lo, C.-H., Lee, T.-Y.
(Eds.), Mantle Dynamics and
Plate Interactions in East Asia, American Geophysical Union, Washington,
DC, pp. 107–126.
-
Herzberg, C., 2006. Petrology
and thermal structure of the Hawaiian plume from
Mauna Kea volcano. Nature 444, 605–609.
-
Hofmann,
A.W., White,W.M., 1982. Mantle plumes from ancient
oceanic crust. Earth Planet.
Sci. Lett. 57, 421–436.
-
Huang, F., Li, S., Yang, W.,
2007. Contributions of the lower crust to Mesozoic
mantle-derived mafic rocks from the North China
Craton: implications for lithospheric thining.
In: Zhai, M.-G., Windley, B.F., Kusky,
T.M., Meng, Q.R. (Eds.), Mesozoic
Sub-Continental Lithospheric Thining under Eastern
Asia. Geol.
Soc. London Spec. Pub., vol. 280, pp. 55–75.
-
Jull,
M., Kelemen, P.B., 2001. On the conditions for
lower crustal convective instability. J.
Geophys. Res. 106, 6423–6446.
-
Kay, R.W., Kay, S.M., 1991.
Creation and destruction of lower continental crust.
Geol. Rundschau 80, 259–278.
-
Kogiso,
T., Hirschmann, M.M., 2001. Experimental study
of clinopyroxenite partial melting and the origin
of ultra-calcic melt inclusions. Contrib.
Mineral. Petrol. 142, 347–360.
-
Kogiso, T., Hirschmann,
M.M., Frost, D.J., 2003. High-pressure partial
melting of garnet pyroxenite: possible mafic lithologies
in the source of ocean island basalts. Earth
Planet. Sci. Lett. 216, 603–617.
-
Lee,
S.Y., Walker, R.J., 2006. Re-Os isotope systematics
of mantle xenoliths from South Korea: Evidence
for complex growth and loss of lithospheric mantle
beneath East Asia. Chem. Geol. 231, 90–101.
-
Liu, D.-Y.,
Nutman, A.P., Compston, W., Wu, J.S., Shen, Q.-H.,
1992. Remnants of >3800 Ma crust
in the Chinese part of the Sino-Korean craton. Geology 20, 339–342.
-
Lustrino, M., 2005. How the
delamination and detachment of lower crust can
influence basaltic magmatism. Earth-Sci.
Rev. 72,
21–38.
-
McKenzie, D., O'Nions,
R.K, 1983. Mantle reserviors and oceanic basalts.
Nature 301, 229-231.
-
Menzies,
M.A., Fan, W.-M., Zhang, M., 1993. Paleozoic and
Cenozoic lithoprobes and the loss of >120
km of Archean lithosphere, Sino-Korean craton, China.
In: Prichard, H.M., Alabaster, T., Harris,
N.B.W., Neary, C.R. (Eds.), Magmatic Processes
and Plate Tectonics. Geol.
Soc. London Spec. Pub.,
vol. 76, pp. 71–81.
-
Menzies, M.A., Xu, Y., Zhang,
H., Fan, W., 2007. Integration of geology, geophysics
and geochemistry: A key to understanding the North
China Craton. Lithos 96, 1–21.
-
Rapp, R.P., Shimizu, N., Norman,
M.D., Applegate, G.S., 1999. Reaction between slab-derived
melts and peridotite in the mantle wedge: experimental
constraints at 3.8 GPa. Chem.
Geol. 160, 335–356.
-
Rudnick,
R.L., Gao, S., Ling, W.L., Liu, Y.S., McDonough,
W.F., 2004. Petrology and geochemistry of spinel
peridotite xenoliths from Hannuoba and Qixia, North
China Craton. Lithos 77, 609–637.
-
Skjerlie,
K.P., Patino Douce, A.E., 2002. The fluid-absent
partial melting of a zoisite-bearing quartz eclogite
from 1.0 to 3.2 GPa: Implications for melting in
thickened continental crust and for subduction-zone
processes. J. Petrol. 39, 29–60.
-
Sobolev, A.V.,
Hofmann, A.W., Sobolev, S.V., Nikogosian, I.K.,
2005. An olivine-free mantle source of Hawaiian
shield basalts. Nature 434, 590–597.
-
Sobolev,
A.V., et al., 2007. The amount of recycled crust
in sources of mantle-derived melts. Science 316,
412–417.
-
Thompson, R.N., Gibson, S.A.,
2000. Transient high temperatures in mantle plume
heads inferred from magnesian olivines in Phanerozoic
picrites. Nature 407, 502–506.
-
Wu, F.Y., Walker,
R.J., Ren, X.W., Sun, D.Y., Zhou, X.H., 2003. Osmium
isotopic constraints on the age of lithospheric
mantle beneath northeastern China. Chem.
Geol. 197, 107-129.
-
Wu, F.Y., Lin, J.Q., Wilde,
S.A., Zhang, X.O., Yang, J.H., 2005. Nature and
significance of the Early Cretaceous giant igneous
event in eastern China. Earth
Planet. Sci. Lett. 233, 103–119.
-
Wu, F.Y.
Walker, R.J., Yang, Y.H., Yuan, H.L., Yang, J.
H., 2006. The chemical-temporal evolution of lithospheric
mantle underlying the North China Craton. Geochim.
Cosmochim. Acta 70, 5013–5034.
-
Xu, W.L., Gao,
S. Wang, Q.-H., Wang, D.Y., Liu, Y.S., 2006. Mesozoic
crustal thickening of the eastern North China Craton:
Evidence from eclogite xenoliths and petrologic
implications. Geology 34, 721–724.
-
Xu,
W.L., Hergt, J.M., Gao, S., Pei, F.P., Wang, W.,
Yang, D.P., 2008. Interaction of adakitic melt-peridotite:
Implications for the high-Mg# signature of Mesozoic
adakitic rocks in the eastern North China Craton.
Earth Planet. Sci. Lett. 265, 123-137.
-
Xu, Y.G.,
2001. Thermo-tectonic destruction of the Archean
lithospheric keel beneath the Sino-Korean Craton
in China: Evidence, timing and mechanism. Phys.
Chem. Earth (A) 26, 747–757.
-
Yaxley, G.M.,
2000. Experimental study of the phase and melting
relations of homogeneous basalt plus peridotite
mixtures and implications for the petrogenesis
of flood basalts. Contrib.
Mineral. Petrol. 139,
326–338.
-
Yaxley, G.M., Green, D.H., 1998.
Reactions between eclogite and peridotite: mantle
refertilisation by subduction of oceanic crust.
Schweiz. Mineral. Petrogr.
Mitt. 78, 243–255.
-
Zhang, H.-F., Ying, J.-F.,
Shimoda, G., Kita, N.T., Morishita, Y., Shao, J.-A.,
Tang, Y.-J., 2007. Importance of melt circulation
and crust-mantle interaction in the lithospheric
evolution beneath the North China Craton: Evidence
from Mesozoic basalt-borne clinopyroxene xenocrysts
and pyroxenite xenoliths. Lithos 96, 67–89.
-
Zhang,
H.-F., Goldstein,
S.L., Zhou,
X.H., Sun,
M., Zheng,
J.-P., Cai,
Y., 2008. Evolution
of subcontinental lithospheric mantle beneath
eastern China: Re–Os isotopic
evidence from mantle xenoliths in Paleozoic kimberlites
and Mesozoic basalts.
Contrib. Mineral. Petrol. 155, 271-293.
-
Zhao, G.C., Sun, M., Wilde,
S.A., Li, S.Z., 2005. Late Archean to Paleoproterozoic
evolution of the North China Craton: key issues
revisited. Precambrian Res. 136, 177–202.
-
Zheng, J.P. Griffin, W.L.,
O'Reilly, S.Y., Lu, F.X., Wang, C.Y., Zhang, M.,
Wang, F.Z., Li, H.M., 2004. 3.6 Ga lower crust
in central China: new evidence on the assembly
of the North China Craton. Geology 32, 229–232.
-
Zheng, J.P., Griffin, W.L.,
O’Reilly,
S.Y., Yang, J.S., Li, T.F., Zhang, M., Zhang, R.Y.,
Liou, J.G., 2006. Mineral chemistry of peridotites
from Paleozoic, Mesozoic and Cenozoic lithosphere:
Constraints on mantle evolution beneath Eastern China.
J. Petrol. 47, 2233–2256.
-
Zhu, R.X., Lo, C.-H., Shi, R.P.,
Shi, G.H., Pan, Y.X., Shao, J., 2004. Palaeointensities determined
from the middle Cretaceous basalt in Liaoning Province,
northeastern China. Phys.
Earth Planet. Inter. 142,
49–59.
last updated 21st
March, 2008 |