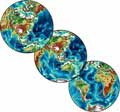 |
No major control by hot-spot-related mantle processes on the evolution of the Laptev Sea, the South China Sea, and the southern South Atlantic |
Dieter
Franke
Federal Institute
for Geosciences and Natural Resources, Hannover, Germany
(BGR), Dieter.Franke@bgr.de
This webpage summarises the key findings of the paper: Franke, D., Rifting, lithosphere breakup and volcanism: Comparison of magma-poor and volcanic rifted margins, Marine and Petroleum Geology (2012), doi: 10.1016/j.marpetgeo.2012.11.003.
Introduction
Traditionally, active rifts are thought to evolve in response to thermal upwelling of the asthenosphere, whereas passive rifts develop in response to lithospheric extension driven by far-field stresses. Depending on the volumes of extrusives produced during rifting, one of two end-member passive margin types may develop–either volcanic or magma-poor.
In a recent study Franke (2012) discussed three unique rifted margins:
-
The Laptev Sea margin in the Arctic Ocean, where the active Arctic mid-oceanic ridge meets continental lithosphere at a high angle,
-
The South China Sea that may represent an intermediate form of continental extension between the end member extremes, and
-
The southernmost South Atlantic with well-expressed conjugate volcanic rifted margins, traditionally interpreted as a result of the Tristan da Cunha mantle plume.
This webpage summarizes key aspects of volcanic rifted margins and magma-poor margins with respect to the role of the mantle, including a description of the stratigraphic response to rifting and continental breakup.
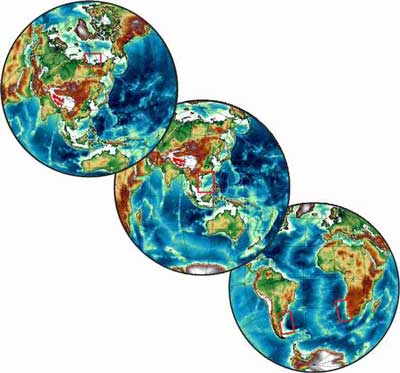
Franke (2012) concluded that rift-plume interactions are not simple. Some volcanic-rifted margins cannot be directly linked to hot spots (van Wijk et al., 2001), and where a spatial link does exist, a wide range in delays between rifting and the impingement of hot spots on zones of crustal extension is observed (Nikishin et al., 2002; Ziegler & Cloetingh, 2004). For the three examples listed above:
-
The presence of a hot spot does not necessarily result in volcanic rifting (Laptev Sea),
-
A hot spot may evolve long after magma-poor rifting and seafloor-spreading (South China Sea), and,
-
A major controlling role for hot-spot-related mantle processes cannot be observed in the Southern Atlantic.
Rifted margins and rift-related magmatism
The recovery of upper-mantle peridotite along the Galicia margin challenged the interpretation of magma-poor margins and drew scientists to develop new models of rifting and continental breakup (Boillot et al., 1995; Karner et al., 2007; Manatschal & Bernoulli, 1999; Péron-Pinvidic & Manatschal, 2009; Reston, 2007; Tucholke & Sibuet, 2007; Whitmarsh et al., 2001). This ultimately resulted in the distinction between volcanic-rifted- and magma-poor margins (Figure 1). The differentiation between these two end-member passive margins thus is mainly based on the volumes of magmas and classification may not always be easy. Along-strike differences in rifts suggest a continuum between end-members.
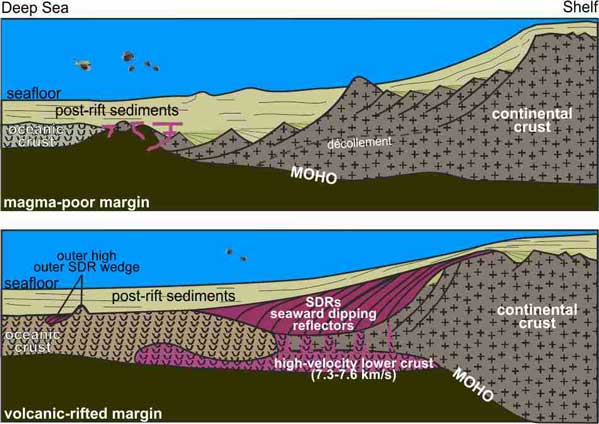
Figure 1: End-member extremes of passive continental margins.
At magma-poor margins (Figure 1) the shelf region is characterized by high-angle listric faults related to fault-bounded rift basins, while the slope area is characterized by extremely thinned crust that potentially is separated from the oceanic crust by a domain of exhumed subcontinental mantle. The boundary between the two domains is the point where the listric faults penetrate the entire crust to the mantle. This leads to coupling and the development of a detachment at the crust-mantle boundary, allowing the exhumation of mantle further seaward. At the time of breakup, with the onset of seafloor spreading, magmatism becomes increasingly important at magma-poor margins, and is mainly close to the seaward limit of continental crust.
Volcanic rifted margins (Figure 1) are characterized by thick wedges of volcanic flows (Hinz, 1981; Mutter et al., 1982), manifested in multichannel seismic reflection data as seaward dipping reflectors (SDRs) and high-velocity (Vp > 7.3 km/s) lower crust, seaward of the continental rifted margin (Eldholm et al., 1987; Menzies et al., 2002; Roberts et al., 1984; Skogseid, 2001). Because the quick generation of voluminous amounts magma requires large and rapid amounts of melting in the mantle, White (1989) proposed that an anomalously hot mantle (150-200° above normal) must be present under the rift shortly before continental breakup to enable the formation of volcanic rifted margins. Subsequently it has been proposed that either such temperature anomalies, or mantle plumes by themselves, cause the breakup of continents (e.g., Richards et al., 1989), or that hot material accumulates at the base of the lithosphere so that lithospheric thinning and decompression melting during rifting generates much greater amounts of magma than over mantle of normal temperature (White & McKenzie, 1989). The magmatism associated with the North Atlantic volcanic rifted margins thus was proposed to have been caused by passive upwelling and decompression melting of asthenospheric mantle, with the hot mantle introduced by the initiation of the Iceland plume (White, 1989).
Recently, studies in different disciplines found data which do not agree well with this “plume concept” and currently there is considerable controversy over the mechanism of production of large volumes of basaltic magmatism. In this context I discuss below three unique rifted margins that are infrequently cited in the literature.
The Laptev Sea Rift
The Laptev Sea rift (Figure 2) is a modern example of initial continental breakup. It occupies the North-Eastern Siberian shelf region, where the active mid-ocean spreading ridge (the Gakkel Ridge) meets the slope of a continental margin. The tectonic and structural evolution of the Laptev Sea Rift System is probably related to opening of the Eurasia Basin and the evolution of the Gakkel spreading ridge. Rifting, which probably initiated in the Late Cretaceous – earliest Cenozoic, was magma-poor (Drachev et al., 1998; Franke & Hinz, 2005; Franke et al., 2001). Also the major parts of the New Siberian Islands, except the De Long Islands are reported to lack extrusive magmatism (Dorofeev et al., 1999).
The De Long Province (Figure 2) is unusual concerning magnetic field data. Potentially rift-related basalts are widespread on these islands where the flows make up two 100-m successions (Figure 2). The basalts have an intraplate geochemical signature, and K–Ar whole-rock ages constrain the age of the basaltic magmatism to the Aptian/Albian stage (Dorofeev et al., 1999; Drachev & Saunders, 2006; Kuzmichev, 2009). In the area of the De Long Islands there are also Paleozoic and Neogene-Quaternary basalts (Dorofeev et al., 1999). The De Long volcanic province thus may be considered the surface expression of a potentially long-lived hot spot. This hot spot lay at the edge of the future Laptev Sea Rift System, and was most active around initial rifting times. It was thus an excellent candidate to deliver breakup-related basalts. However, it apparently was not involved in the Laptev Sea rifting process, which developed a magma-poor rift and continental margin.
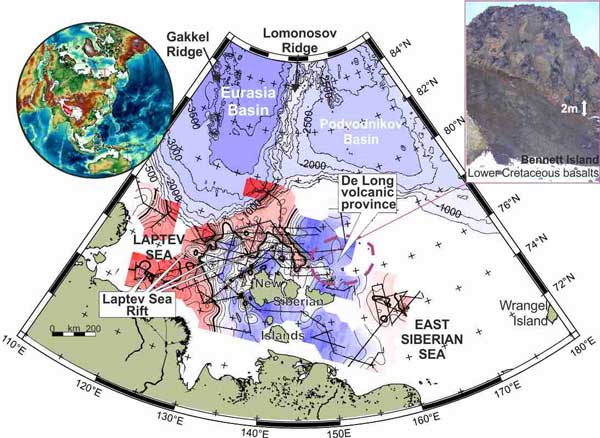
Figure 2: Map showing the depth of sedimentary cover to the acoustic basement based on multichannel seismic lines. Rift basins are shown in red/pink. The photography shows thick, Late Lower Cretaceous basalts resting on Ordovician turbitites on Bennett Island, within the De Long volcanic province.
The South China Sea
The South China Sea (Figure 3) is one of the largest marginal seas in the western Pacific. In addition to its oceanic basin, the South China Sea includes a domain of highly attenuated continental crust which is similar in area to the Basin and Range province in the western USA (> 106 km2).
The earliest phase of rifting in the South China Sea started in the Late Cretaceous to Early Paleocene when a Mesozoic convergent margin changed to extension. Rifting and subsequently Oligocene to Early Miocene seafloor-spreading migrated from the NE to the SW. The South China Sea, with its V-shaped oceanic basin, is clearly a propagator bordered by magma-poor margins (Clift et al., 2001; Franke et al., 2011; Hu et al., 2009). With the cessation of seafloor-spreading in the Early Miocene volcanism became increasingly active (Flower et al., 1992; Yan et al., 2006). Widespread post-rift volcanism is manifest in distinctive seamounts up to 4 km high that are aligned along the extinct spreading axis, and transform faults and numerous other volcanoes around the ocean basin (Figure 3).
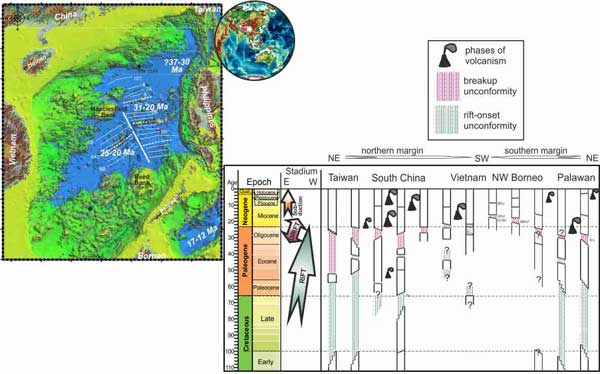
Figure 3: Topography and bathymetry of the South China Sea region and chronology of tectonism around the South China Sea. The area of highly attenuated continental crust (yellow/light green) is > 1.000 km wide. Click here or on Figure for enlargement.
Whether the scattered Cenozoic magmatism over and around the South China Sea and its adjacent areas shares a common origin is unclear. However, tomographic images of S-wave velocity confirm the existence of a mantle plume, the ‘Hainan Plume’. Montelli et al. (2006) proposed a deep mantle origin from a weak but resolved velocity perturbation which is visible down to about 1900 km depth under Hainan. [Ed: See also Banana Doughnut page].
The major magmatic expulsion of this ‘Hainan Plume’ took place from the Pliocene onwards, about 10 Ma after the cessation of seafloor-spreading and more than 20 Ma after the end of the main magma-poor rift phase. This is therefore another example with no unequivocal link between an onshore flood basalt province that is related to a hot spot and the volume of magmatism during rifting.
The Southern Atlantic and the Tristan da Cunha plume
West Gondwana broke up in Early Cretaceous times and subsequent seafloor spreading resulted in the formation of the South Atlantic Ocean. Continental breakup and initial seafloor spreading in the South Atlantic were accompanied by extensive transient magmatism as inferred from sill intrusions, flood basalt sequences, voluminous volcanic wedges, and high-velocity lower crust at the present continental margins (Figure 4). These voluminous basaltic sequences are conventionally related to the Tristan da Cunha hot spot.
This hot spot is one of the rare examples meeting the characteristics of a deep plume (Courtillot et al., 2003). [Ed: See also Plates vs. Plumes Section 1.5.] It reveals flood-basalt magmatism at its initiation and long-lived volcanic tracks expressed as the largest bathymetric features of the South Atlantic, the Walvis Ridge and the Rio Grande Rise. However, the seismic anomaly beneath the Tristan da Cunha hot spot is consistently found to be confined to the upper mantle (Foulger, 2005).
It is a popular opinion that the mid-Cretaceous opening of the southern South Atlantic was initiated and predated by the extrusion of the Etendeka and Paraná flood basalts (e.g., Gladczenko et al., 1997; Wilson, 1992). However, there are indications that near the Paraná-Etendeka flood basalts rifting preceded emplacement of the flood basalts, that the latter were derived mainly from the shallow mantle, and that a number of structural findings conflict with the plume-initiated-breakup model.
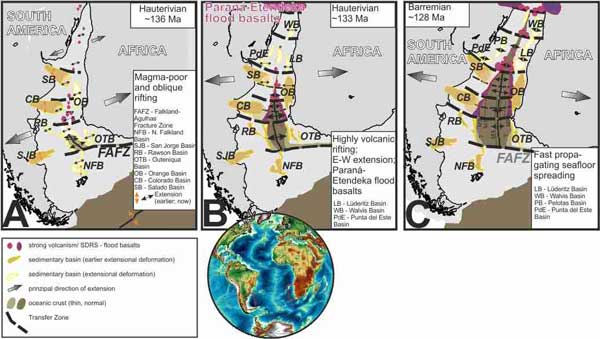
Figure 4: The early evolution of the southern South Atlantic at (A) ca. 137 Ma, (B) ca. 133 Ma and (C) ca. 128 Ma. (A) Extension coincident with subsequent seafloor spreading following oblique and magma-poor rifting in the southernmost segment initiated in the late Valanginian or early Hauterivian. Probably there was already major volcanism at the future volcanic rifted margins, as shown by the SDRs. (B) The segment to the north of the transfer zone, defining the transition from magma-poor to volcanic rifting opens at about 133 Ma. At the next transfer zones further north, rifting is interrupted, resulting in heat accumulation in the upper mantle. This enhances convection in the asthenosphere and the subsequent emplacement of multiple SDR wedges and the emplacement of the Paraná-Etendeka flood basalts between mainly between 134 Ma and 132 Ma. (C) After stepping across the next segment boundary, or transfer zone, seafloor spreading propagated quickly north, reaching the transfer zone that bounds the southern to the central South Atlantic by Barremian time. Again heat accumulation and enhanced mantle convection is proposed as for the southernmost margin, resulting in the emplacement of multiple SDR wedges in this margin segment. Click here or on Figure for enlargement.
First, there is no spatial relationship. The South Atlantic started opening at its southernmost point, close to the present Falkland-Agulhas Fracture Zone (Figure 4). At this time, the hot spot was more than 2.000 km further north. Second, the timing is not appropriate. The vast majority of the Etendeka and Paraná flood basalts were emplaced when there was already ongoing seafloor spreading in the south (Figure 4). Thus, the hot spot became involved when rifting had already ceased in the south. It can be argued that the plume head already underlay the rift without major extrusions. However, the sharp transition from magma-poor to volcanic rifting as observed at the southern Argentine margin (see Argentina page; Franke et al., 2010) argues against a deep mantle origin for the rift-related magmatism. A plume rising from the deep mantle is expected to generate a smooth transition from magma-starved to volcanic rifting over a few hundreds of kilometers rather than a few tenths of a kilometers. A relationship between the proposed hot spot and the volcanic margins thus seems doubtful.
Possible mechanisms for volcanic rifting
Passive continental margins are so diverse that the development of generalized models is not simple. Further research will improve understanding of how the volume of rift-related magmatism depends on the pre-rift configuration and the rift history, and to what degree elevated mantle temperatures are necessary to explain volcanic margins. Here I present some thoughts about rift-hot spot interactions based on the three example passive margins described above.
A major controlling role for deep, hot-spot-related mantle processes on rift evolution and rift-related magmatism cannot be observed (Franke, 2012). Instead, it is suggested that passive margin evolution is controlled by lithosphere-scale processes and parameters, including rift propagation and propagation barriers.
In the Laptev Sea region, a close spatial relationship between a rift and a hot spot did not result in volcanic rifting. Therefore there is no observed link between onshore flood basalt magmatism, a hot spot, and magmatic volume during rifting.
In the South China Sea, the Hainan Plume developed late, after cessation of seafloor-spreading. The termination of seafloor-spreading may, in fact, offer an explanation for the “plume volcanism”. Perhaps a well-established mantle convection system, supplying melt to form oceanic crust, continued after spreading suddenly ceased. The melt then found other ways to the surface, forming volcanic cones, seamounts and volcanic flows. The position of the Hainan Plume, centered beneath the extinct spreading ridge, may suggest that the hot spot volcanism originated in this way.
Might a similar process explain the Tristan da Cunha hot spot? Seafloor-spreading was delayed across the southern-to-central segments of the South Atlantic. The Brazil-Angola margins of the central segment are extremely extended, suggesting a much longer rift phase before breakup. Rift propagation was delayed, possibly due to the presence of more rigid Gondwana-core basement. The concept of rift-propagation barriers, suggested to enhance the supply of melt from decompressional melting the other side of the structural discontinuity, (see Argentina page; Franke et al., 2007), is thus is in accordance with the locations of the Etendeka and Paraná flood basalts. However, in order to explain the long-lived, post-rifting volcanism of the Walvis Ridge and Rio Grande Rise, it would be necessary to assume that this extension-induced hot spot continued to develop with time, perhaps growing downwards from its originial, shallow form. It may have progressively incorporated melt from the lower lithosphere, and perhaps also the asthenosphere, and fed the time-progressive volcanism. Nevertheless, the hot spot was not the origin of volcanic rifting, nor the along-margin intrusive and extrusive magmatism, and the SDRs.
Such a model may be applicable to other areas, as many hot spots are apparently located at positions where rift could not propagate straight forward, e.g., at triple junctions. An intrinsic relationship between mantle plumes and triple junctions was proposed by Burke & Dewey (1973) for 45 selected junctions. Such a link may exist but, contrary to this study, I speculate that hot spots did not generate the junctions but that hot spots may have developed where rift-propagation was hindered.
References
- Boillot, G., Beslier, M.O., Krawczyk, C.M., Rappin, D., Reston, T.J., 1995. The formation of passive margins: Constraints from the crustal structure and segmentation of the Galicia margin, Spain, in: Scrutton, R.A., Stoker, M.S., Shimmield, G.B., Tudhope, A.W. (Eds.), The tectonics, sedimentation and paleooceanography of the North Atlantic region. Geological Society of London, Special Publication, London, pp. 71-91.
- Burke, K., Dewey, J.F., 1973. Plume-generated tripple junctions: Key indicators in applying plate tectonics to old rocks. Journal of Geology 81, 406-433.
- Clift, P.D., Lin, J., ODP Leg 184 Scientific, P., 2001. Patterns of extension and magmatism along the continent-ocean boundary, South China margin. Geological Society, London, Special Publications 187, 489-510.
- Courtillot, V., Davaille, A., Besse, J., Stock, J., 2003. Three distinct types of hotspots in the Earth's mantle. Earth and Planetary Science Letters 205, 295-308.
- Dorofeev, V.K., Blagoveshchensky, M.G., Smirnov, A.N., Ushakov, V.I., 1999. New Siberian Island. Geological structure and metallgeny. VNIIOkeangeologia.
- Drachev, S., Saunders, A.D., 2006. The Early Cretaceous Arctic Lip: Its geodynamic setting and implications for Canada Basin opening, in: Scott, R.A., Thurston, D.K. (Eds.), Proceedings of the Fourth International Conference on Arctic Margins ICAM IV. US Department of the Interior, pp. 216-223.
- Drachev, S.S., Savostin, L.A., Groshev, V.G., Bruni, I.E., 1998. Structure and geology of the continental shelf of the Laptev Sea, Eastern Russian Arctic. Tectonophysics 298, 357-393.
- Eldholm, O., Thiede, J., Taylor, B., 1987. Proc. ODP, Sci. Results. College Station, TX (Ocean Drilling Program).
- Flower, M.F.J., Zhang, M., Chen, C.-Y., Tu, K., Xie, G., 1992. Magmatism in the South China Basin: 2. Post-spreading Quaternary basalts from Hainan Island, south China. Chemical Geology 97, 65-87.
- Foulger, G.R., 2005. Mantle plumes: Why the current skepticism? Chinese Science Bulletin 50, 1555-1560
- Franke, D., Rifting, lithosphere breakup and volcanism: Comparison of magma-poor and volcanic rifted margins, Marine and Petroleum Geology (2012), doi: 10.1016/j.marpetgeo.2012.11.003.
- Franke, D., Barckhausen, U., Baristeas, N., Engels, M., Ladage, S., Lutz, R., Montano, J., Pellejera, N., Ramos, E.G., Schnabel, M., 2011. The continent-ocean transition at the southeastern margin of the South China Sea. Marine and Petroleum Geology 28, 1187-1204.
- Franke, D., Hinz, K., 2005. The structural style of sedimentary basins on the shelves of the Laptev Sea and the western East Siberian Sea, Siberian Arctic. Journal of Petroleum Geology 28, 269-286.
- Franke, D., Hinz, K., Oncken, O., 2001. The Laptev Sea Rift. Marine and Petroleum Geology 18, 1083-1127.
- Franke, D., Ladage, S., Schnabel, M., Schreckenberger, B., Reichert, C., Hinz, K., Paterlini, M., de Abelleyra, J., Siciliano, M., 2010. Birth of a volcanic margin off Argentina, South Atlantic. Geochem. Geophys. Geosyst. 11, Q0AB04.
- Franke, D., Neben, S., Ladage, S., Schreckenberger, B., Hinz, K., 2007. Margin segmentation and volcano-tectonic architecture along the volcanic margin off Argentina/Uruguay, South Atlantic. Marine Geology 244, 46-67.
- Gladczenko, T.P., Hinz, K., Eldholm, O., Meyer, H., Neben, S., Skogseid, J., 1997. South Atlantic volcanic margins. Journal of the Geological Society 154, 465-470.
- Hinz, K., 1981. A hypothesis on terrestrial catastrophes: wedges of very thick oceanward dipping layers beneath passive continental margins—their origin and paleoenvironmental significance. Geologisches Jahrbuch Reihe E, 3-28.
- Hu, D., Zhou, D., Wu, X., He, M., Pang, X., Wang, Y., 2009. Crustal structure and extension from slope to deepsea basin in the northern South China Sea. Journal of Earth Science 20, 27-37.
- Karner, G.D., Manatschal, G., Pinheiro, L.M., 2007. Imaging, mapping and modelling continental lithosphere extension and breakup: an introduction. Geological Society, London, Special Publications 282, 1-8.
- Kuzmichev, A.B., 2009. Where does the South Anyui suture go in the New Siberian islands and Laptev Sea?: Implications for the Amerasia basin origin. Tectonophysics 463, 86-108.
- Manatschal, G., Bernoulli, D., 1999. Architecture and tectonic evolution of nonvolcanic margins: Present day Galicia and ancient Adria. Tectonics 18, 1099-1119.
- Menzies, M.A., Klemperer, S.L., Ebinger, C.J., Baker, J., 2002. Characteristics of volcanic rifted margins, in: Menzies, M.A., Klemperer, S.L., Ebinger, C.J., Baker, J. (Eds.), Volcanic Rifted Margins. Geological Society of America Special Paper Boulder, Colorado, pp. 1-14.
- Montelli, R., Nolet, G., Dahlen, F.A., Masters, G., 2006. A catalogue of deep mantle plumes: New results from finite-frequency tomography. Geochem. Geophys. Geosyst. 7, Q11007.
- Mutter, J.C., Talwani, M., Stoffa, P.L., 1982. Origin of seaward-dipping reflectors in oceanic crust off the Norwegian margin by "subaerial sea-floor spreading". Geology 10, 353-357.
- Nikishin, A.M., Ziegler, P.A., Abbott, D., Brunet, M.F., Cloetingh, S., 2002. Permo–Triassic intraplate magmatism and rifting in Eurasia: implications for mantle plumes and mantle dynamics. Tectonophysics 351, 3-39.
- Péron-Pinvidic, G., Manatschal, G., 2009. The final rifting evolution at deep magma-poor passive margins from Iberia-Newfoundland: a new point of view. International Journal of Earth Sciences 98, 1581-1597.
- Reston, T.J., 2007. The formation of non-volcanic rifted margins by the progressive extension of the lithosphere: the example of the West Iberia margin, in: Karner, G., Manatschal, G., Pinheiro, L.D. (Eds.), Imaging, Mapping and Modelling Continental Lithosphere Extension and Breakup. Geological Society of London Special Publication, London, pp. 77-110.
- Richards, M.A., Duncan, R.A., Courtillot, V.E., 1989. Flood Basalts and Hot spot Tracks: Plume Heads and Tails. Science 246, 103-107.
- Roberts, D.G., Backman, J., Morton, A.C., Murray, J.W., Keene, J.B., 1984. Evolution of volcanic rifted margins: Synthesis of Leg 81 results on the west margin of Rockall Plateau, Initial Reports of the Deep Sea Drilling Project 81. Texas A & M University, Ocean Drilling Program, College Station, TX, United States, pp. 883-911.
- Skogseid, J., 2001. Volcanic margins: geodynamic and exploration aspects. Marine and Petroleum Geology 18, 457-461.
- Tucholke, B.E., Sibuet, J.-C., 2007. Leg 210 Synthesis: Tectonic, Magmatic, and Sedimentary Evolution of the Newfoundland-Iberia Rift, in: Tucholke, B.E., Sibuet, J.-C., Klaus, A. (Eds.), Proceedings of the Ocean Drilling Program, Scientific Results, College Station, TX, pp. 1-56.
- van Wijk, J.W., Huismans, R.S., ter Voorde, M., Cloetingh, S.A.P.L., 2001. Melt generation at volcanic continental margins: No need for a mantle plume? Geophysical Research Letters 28, 3995-3998.
- White, R., McKenzie, D., 1989. Magmatism at Rift Zones: The Generation of Volcanic Continental Margins and Flood Basalts. Journal of Geophysical Research 94, 7685-7729.
- White, R.S., 1989. Initiation of the Iceland plume and opening of the North Atlantic. Extensional tectonics and stratigraphy of the North Atlantic margins, AAPG Special Volume A156, 149-154.
- Whitmarsh, R.B., Manatschal, G., Minshull, T.A., 2001. Evolution of magma-poor continental margins from rifting to seafloor spreading. Nature 413, 150-154.
- Wilson, M., 1992. Magmatism and continental rifting during the opening of the South Atlantic Ocean: a consequence of Lower Cretaceous super-plume activity? , in: Storey, B.C., Alabaster, T., Pankhurst, R.J. (Eds.), Magmatism and the Causes of Continental Break-up. Spec. Publ.-Geol. Soc. London, London, pp. 155- 241.
- Yan, P., Deng, H., Liu, H., Zhang, Z., Jiang, Y., 2006. The temporal and spatial distribution of volcanism in the South China Sea region. Journal of Asian Earth Sciences 27, 647-659.
- Ziegler, P.A., Cloetingh, S., 2004. Dynamic processes controlling evolution of rifted basins. Earth-Science Reviews 64, 1-50.
last updated 12th February, 2013 |