 |
|
Definition of a Cenozoic alkaline magmatic
province in the southwest Pacific mantle
domain and without rift or plume origin
|
|
Carol A. Finn1, R.
Dietmar Müller2
& Kurt
S. Panter3
1U.S.
Geological Survey, MS 964, Denver Federal Center,
Denver, CO 80225, USA
cfinn@usgs.gov
2School
of Geosciences, Edgeworth David Bldg. F05, The University
of Sydney, 2006, AUSTRALIA
dietmar@geosci.usyd.edu.au
3Department
of Geology, Bowling Green State University, Bowling
Green, OH
43403-0218, USA
kpanter@bgnet.bgsu.edu
|
A Cenozoic (< 50 Ma) bimodal,
but largely basaltic, mostly alkaline igneous province
covers a broad area of continental and oceanic lithosphere
in the southwest Pacific Ocean region (Figure 1).
It has been conjecturally linked to rifting, mantle
plumes, or hundreds of hot spots, but all of these
associations have flaws. For example, plate reconstructions
demonstrate that the last episode of major regional
rifting in west Antarctica, eastern Australia and
New Zealand occurred during the Mesozoic break-up
of Gondwana. GPS and stress-field measurements show
no extension in Australia, New Zealand and much
of west Antarctica, suggesting that the widespread
magmatism cannot be explained by rifting alone.
Estimates of volumes of magmas erupted in west Antarctica
and Australia, as well as magma production rates
are low compared to areas associated with plumes.
Uplift and doming typically associated with mantle
plumes are also largely absent. Also, to explain
the areal distribution of the volcanism, an unusually
large plume would have to underlie the entire southwest
Pacific, or there would have to be hundreds of hot
spots, which are not observed. Clearly, new models
for volcanism are required.
|
Figure 1. Rayleigh wave 150s
group velocity map (~120 km depth) [Larson and Ekström,
2001]. Only long-lived hot spots with long
traces [Clouard and Bonneville, 2001; Gaina et al.,
2000; Ritsema and Allen, 2003] underlain by low
velocity perturbations in the upper mantle are shown.
AAD=Australian-Antarctic Discordance; LHR=Lord Howe
Rise; RS=Ross Sea; TAM=Transantarctic Mountains,
BH=Bellingshausen Sea. Click on image to enlarge.
|
Comparison of the location of volcanoes and seismic
shear wave perturbation models (Figure 1), shows
that this alkaline volcanic province occurs in thin
(< 80 km) lithosphere [Ritzwoller et al.,
2001] (e.g., Figure 2a). The province correlates
with distinct low seismic velocity anomalies (Figure
1) generally restricted to a zone in the mantle
between ~ 60 and 200 km depth (e.g., Figure 2a).
In places, low velocity perturbations (> 1.2%
decrease from PREM), such as those beneath the Lord
Howe Rise (Figure 2b), extend to ~ 670 km depth
and the Bellingshausen Sea to ~ 800 km depth (Figure
2c). The prominent lower mantle central Pacific
low velocity perturbation zone (Figure 2b) does
not seem to penetrate the 670 km discontinuity [Ritsema
& Allen, 2003; Su et al., 1994]
nor extend south to the Antarctic region (Figure
2c). All of these examples show that the low velocity
anomaies in the region, generally interpreted to
arise from high temperatures, are restricted to
the upper mantle.
|
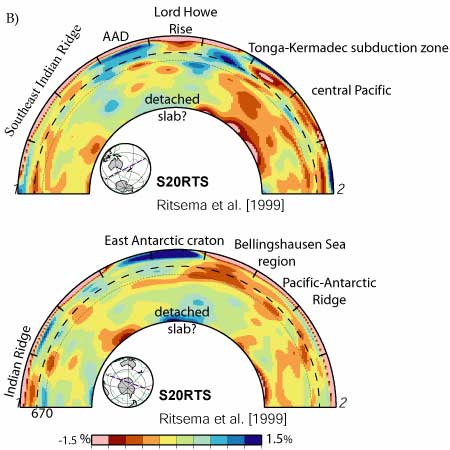
Figure 2. A) (left) Shear wave velocity perturbation
model derived from inversion of Rayleigh
and Love wave data and relative to AK135, Antarctica.
right) Red line shows profile location (from Ritzwoller
et al., 2001).
B) Shear velocity anomalies from model S20RTS
in 180o wide cross-sections through
the mantle [Ritsema et al., 1999]. The thick dashed
line indicates the 670-km discontinuity. (upper)
Section from the Southeast Indian Ridge, across
the Tasman Sea, and Kermadec trench. (lower) Section
from the Indian Ridge, East Antarctic craton,
Bellingshausen Sea, Pacific-Antarctic Ridge and
central Pacific.
|
Geochemical studies show that for most of the region, the
magmatism is a result of small degrees of melting
(F = 1-3%) of a source enriched in incompatible elements
relative to primitive upper mantle. The enrichment
may have involved the introduction of volatile-rich
fluids or melts into pre-existing upper mantle (e.g.,
Gamble et al., 1988; Hart et al.,
1997; Kamenetsky et al., 2000; Panter
et al., 2000; Rocchi et al., 2002; Zhang
et al., 1999). This suggests that melting of
metasomatized upper mantle can occur without excessive
temperatures (Figure 3) and that the low seismic velocities
are primarily related to slightly elevated temperatures,
water and in places, melt. |
Figure 3. McMurdo/SE Australia geotherm (from Berg
et al., 1989; O'Reilly and Griffin, 1985), compared
to stability fields of amphibole and phlogopite.
Also shown are water-saturated and water-undersaturated
solidi and an adiabatic path for asthenospheric mantle. |
The
principal characteristics of alkaline magmatism in
the SW Pacific must be identified for input into any
new models. Striking features of the alkaline province
are its longevity (~ 50 Ma) and broad regional extent
but low volumes. A primary attribute is the low velocity
zone lying between ~ 60 and 200 km depth beneath the
region of alkaline magmatism. Lithospheric thickness
may play a fundamental role in localizing magmatism
in that the volcanism does not occur in regions whose
high velocity lids exceed ~ 80 km. We therefore characterize
the province based on coincident thin lithosphere
hosting largely alkaline magmatism generated from
a metasomatized source associated with low seismic
velocity anomalies in the upper mantle (black line,
Figure 1). The age of the metasomatism is not known
but may relate to a combination of Paleozoic-Mesozoic
subduction along the Pacific margin of Gondwana and
possible plume-related activity in the Jurassic. During
Cretaceous break-up of Gondwana, rifting in east Australia
and west Antarctica did not result in voluminous magmatism
despite thinning and regional extension of continental
lithosphere containing metasomatized mantle. This
suggests that late Cretaceous-early Eocene regional
heating and/or a mantle stirring event is required
to allow alkaline magmatism.
Any satisfactory scenario for generation of the SW
Pacific alkaline magmatic province must explain the
following: 1) broad extent, 2) location in the
SW Pacific, 3) low volumes, 4) HIMU-EM1-EM2 signature,
5) duration, 6) timing of onset, and 7) coincidence
with volcanic centers with linear age progressions
that track plate motion (e.g., the Louisville Ridge,
Tasmintid, Lord Howe and Tasmanian seamounts). Localization
of specific volcanic centers by pre-existing zones
of weakness clearly occurs, but does not seem to drive
the system. Several explanations for episodic plate
reorganization (Fukao et al., 2001; King
et al., 2002) may also provide mechanisms for
mantle flow sufficient to cause alkaline magmatism
in thin, metasomatized lithosphere. Plate motion history
(Lithgow-Bertelloni & Richards, 1998)
and seismic tomography studies (Fukao et al.,
2001; van der Hilst et al., 1997) propose
that high density and velocity subducted slabs lying
in the lower mantle detached from the mantle transition
zone at various times. These slab detachment events,
so-called mantle avalanches, can cause vertical and
lateral flow in the entire mantle (Brunet &
Machetel, 1998; Christensen, 1997; Pysklywec
et al., 2003; Solheim & Peltier,
1994). Detachment of the subducting Pacific slab (now
partially entrained in the AAD) beneath Australia
and Antarctica by the late Cretaceous (~ 65 Ma) (Lithgow-Bertelloni
& Richards, 1998) may have produced viscous
flow of warm mantle to the region. |
References
-
Berg, J.H., R.J. Moscati,
and D.L. Herz, A petrologic geotherm from a continental
rift in Antarctica, Earth and Planetary Science
Letters, 93 (1), 98-108,
1989.
-
Brunet, D., and P. Machetel,
Large-scale tectonic features induced by mantle
avalanches with phase, temperature, and pressure
lateral variations of viscosity, Journal of
Geophysical Research, B, Solid Earth and Planets,
103 (3), 4929-4945, 1998.
-
Christensen, U.R., Influence
of chemical buoyancy on the dynamics of slabs
in the transition zone, Journal of Geophysical
Research, B, Solid Earth and Planets, 102
(10), 22,435-22,443, 1997
-
Clouard, V., and A. Bonneville,
How many Pacific hotspots are fed by deep-mantle
plumes?, Geology, 29
(8), 695-698, 2001.
-
Fukao, Y., S. Widiyantoro,
and M. Obayashi, Stagnant slabs in the upper and
lower mantle transition region, Reviews of
Geophysics, 39 (3), 291-323,
2001.
-
Gaina, C., R.D. Mueller, and
S.C. Cande, Absolute plate motion, mantle flow,
and volcanism at the boundary between the Pacific
and Indian Ocean mantle domains since 90 Ma, in
The History and Dynamics of Global Plate Motions,
edited by M.A. Richards, R.G. Gordon, and
R.D. van der Hilst, pp. 189-210, American Geophysical
Union, Washington, DC, 2000.
-
Gamble, J.A., F. McGibbon,
P.R. Kyle, M.A. Menzies, and I. Kirsch, Metasomatised
xenoliths from Foster Crater, Antarctica; implications
for lithospheric structure and processes beneath
the Transantarctic Mountain front, in Journal
of Petrology, vol.1988, edited by M.A. Menzies,
and K.G. Cox, pp. 109-138, Clarendon Press,
Oxford, 1988.
-
Hart, S.R., J. Blusztajn, W.E.
LeMasurier, and D.C. Rex, Hobbs Coast Cenozoic
volcanism; implications for the West Antarctic
rift system, Chemical Geology, 139,
223-248, 1997.
-
Kamenetsky, V.S., J.L. Everard,
A.J. Crawford, R. Varne, S.M. Eggins, and R.
Lanyon, Enriched end-member of primitive MORB
melts; petrology and geochemistry of glasses
from Macquarie Island (SW Pacific), Journal
of Petrology, 41
(3), 411-430, 2000.
-
King, S.D., J.P. Lowman, and
C.W. Gable, Episodic tectonic plate reorganizations
driven by mantle convection, Earth and Planetary
Science Letters, 203, 83-91,
2002
-
Larson, E.W.F., and G. Ekström,
Global Models of Surface Wave Group Velocity,
Pure & Appl. Geophys, 158
(8), 1377-1400, 2001.
-
Lithgow-Bertelloni, C., and
M.A. Richards, The dynamics of Cenozoic and Mesozoic
plate motions, Reviews of Geophysics,
36 (1), 27-78, 1998
-
Panter, K.S., S.R. Hart, P.
Kyle, J. Blusztanjin, and T. Wilch, Geochemistry
of Late Cenozoic basalts from the Crary Mountains:
characterization of mantle sources in Marie Byrd
Land, Antarctica, Chemical Geology, 165,
215-241, 2000.
-
Pysklywec, R.N., J.X. Mitrovica,
and M. Ishii, Mantle avalanche as a driving force
for tectonic reorganization in the southwest Pacific,
Earth and Planetary Science Letters,
209, 29-38, 2003
-
Ritsema, J., and R.M. Allen,
The elusive mantle plume, Earth and Planetary
Science Letters, 207, 1-12,
2003.
-
Ritsema, J., H.-J. van Heijst,
and J.H. Woodhouse, Complex shear wave velocity
structure imaged beneath Africa and Iceland, Science,
286 (5446), 1925-1928, 1999.
-
Ritzwoller, M.H., N.M. Shapiro,
A.L. Levshin, and G.M. Leahy, Crustal and upper
mantle structure beneath Antarctica and surrounding
oceans, Journal of Geophysical Research, B,
Solid Earth and Planets, 106
(12), 30,645-30,670, 2001.
-
Rocchi, S., P. Armienti, M.
D'Orazio, S. Tonarini, J.R. Wijbrans, and G. Di
Vincenzo, Cenozoic magmatism in the western Ross
Embayment: Role of mantle plume versus plate
dynamics in the development of the West Antarctic
Rift System, Journal of Geophysical Research,
107 (B9), 10.1029/2001JB000515,
2002.
-
Solheim, L.P., and W.R. Peltier,
Avalanche effects in phase transition modulated
thermal convection; a model of Earth's mantle,
Journal of Geophysical Research, B, Solid
Earth and Planets, 99 (4),
6997-7018, 1994
-
Su, W.-J., R.L. Woodward, and
A.M. Dziewonski, Degree 12 model of shear-velocity
heterogeneity in the mantle, Journal of Geophysical
Research, 99, 6945-6980,
1994.
-
van der Hilst, R.D., S. Widiyantoro,
and E.R. Engdahl, Evidence for deep mantle circulation
from global tomography, Nature, 386,
578-585, 1997
-
Zhang, M., S.Y. O'Reilly, and
D. Chen, Location of Pacific and Indian mid-ocean
ridge-type mantle in two time slices: Evidence
from Pb, Sr, and Nd isotopes for Cenozoic Australian
basalts, Geology, 27
(1), 39-42, 1999.
last modified 18th July, 2003 |
|
|