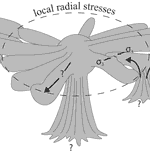 |
Unravelling the complexity of upper crustal dike networks in continental rifts: examples from East Africa |
James D. Muirhead1, Simon A. Kattenhorn1,2 & Nicolas Le Corvec3
1Department of Geological Sciences, University of Idaho, Moscow, Idaho, USA; james.muirhead@fulbrightmail.org ; simon.kattenhorn@gmail.com
2ConocoPhillips Company, Houston, Texas, USA
3Lunar and Planetary Institute, Houston, Texas, USA; lecorvec@lpi.usra.edu
This webpage is a summary of: Muirhead, J. D., S. A. Kattenhorn, and N. Le Corvec (2015), Varying styles of magmatic strain accommodation across the East African Rift, Geochemistry Geophysics Geosystems, 16, doi:10.1002/2015GC005918.
Introduction
The role of dike intrusion in driving continental breakup is fundamental to our understanding of plate tectonics. Buck (2004) showed that the breakup of thick continental lithosphere requires more than far-field tectonic forces, illustrating the important role of dike opening in driving extension during the earliest stages of rifting. Upper crustal diking in rift environments is often depicted to occur through long (up to 80 km), sub-parallel swarms intruding along the full length of rift basins. These assertions are supported by recent dike-driven rifting events in Iceland and Ethiopia (Wright et al., 2012), and inform numerical modelling studies addressing the mechanical effects of dike intrusion to rift processes (e.g., Buck et al., 2005).
Our current view of dike networks, however, may be biased to evolved (>20 Ma), oceanic (e.g., Krafla) or nascent (e.g., Dabbahu-Manda-Hararo) spreading centers. This is largely because magmatic rifting occurs in these regions with a high enough frequency that it can be persistently captured using modern-day monitoring techniques (e.g., InSAR and seismicity). Dike networks throughout other sectors of the East African Rift may instead exhibit greater complexity, particularly in early-stage rifts (<10 Ma) undergoing infrequent diking episodes (Calais et al., 2008). By unravelling the contributions of dikes in these basins we can further refine our understanding of the role of magmatism during continental rift initiation.
Current geophysical techniques (e.g., seismic reflection) lack the capacity to resolve thin, sub-vertical structures in the sub-surface, and thus reconstructing the geometries of cooled, upper crustal dike swarms poses a significant challenge. Recent structural and volcanological studies in both active and eroded monogenetic volcanic fields have illustrated the utility of volcanic vent alignments and cone morphometrics for inferring the distributions and orientations of upper crustal dikes (Kiyosugi et al., 2012; Le Corvec et al., 2013; Keir et al., 2015). The East African Rift exhibits numerous monogenetic cone fields that may help us understand the distribution and geometry of shallow dike-feeders emplaced in the last few million years (Korme et al., 1997; Mazzarini et al., 2013). Building on these studies and methods, we performed a comparative analysis of upper crustal diking in various rift basins throughout East Africa, based on the distributions, alignments and morphologies of monogenetic cones (Muirhead et al., 2015).
How accurate is the conventional model of upper crustal dike systems in continental rifts?
Our recently collected data reveal along-axis variations in the distribution and orientations of dike intrusions in the East African Rift. Rose plots presented in Figure 1 illustrate geometrical variations in select rift basins in Ethiopia, Kenya, Tanzania and the Democratic Republic of Congo (DRC). In evolved basins (>10 Ma) in Kenya and Ethiopia, sub-parallel swarms of upper crustal dikes, similar to classical depictions of dike networks, intrude along the full length of rift basins (Figure 2). However, upper crustal diking in early-stage basins (<10 Ma) in the eastern branch, as well as those across the western branch, diverge from these expected arrangements. In these basins, upper crustal dikes are confined to transfer zones, which are zones of rift-oblique faulting that mechanically link rift segments. Dike orientations are also variable, exhibiting rift-parallel, rift-oblique, and radial patterns (Figure 2).
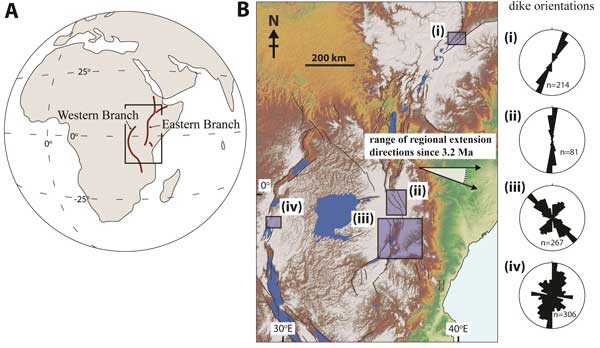
Figure 1: (A) Globe simplifying the overall extent of the eastern and western branches of the East African Rift. Box shows the location of B. (B) Annotated DEM image (90 m Shuttle Radar Topography Mission) of the northeastern portion of the African continent in the black box in A. Faults are shown as black lines and major lakes, which commonly occur in deep rift basins, are colored blue. Rose diagrams show upper crustal dike strikes based on volcanic vent alignments and cone morphometrics. Letters refer to dikes in rift basins in Ethiopia (i), Kenya (ii), Tanzania (iii), and the Democratic Republic of Congo (iv). Figure modified from Muirhead et al. (2015). Click here or on Figure for enlargement.
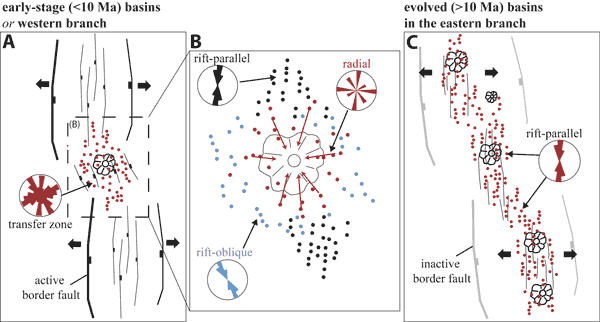
Figure 2: Schematic representation of the distribution of cones and related dike-feeder trends slightly modified from Muirhead et al. (2015). Rose diagrams provide a conceptual example of the expected dike trends based on the structural analyses of cones in different structural settings within basins. Black arrows in A and C represent the regional extension direction (E-W in this example). (A) In early-stage basins and the western branch, cones (red dots) focus in transfer zones and the distal tips of basins. (B) The range of dike-feeder trends distributed within transfer zones in early-stage basins and the western branch. Rift-parallel dikes (black dots, black rose diagram) occur near the boundary between the transfer zone and the distal tip of the basin. Rift-oblique dikes (blue dots, blue rose diagram) occur across the entire transfer zone. Their orientations are controlled by stresses related to the mechanical interaction between rift segments, as well as inherited crustal structure, which together also control the orientation of faults within the transfer zone. Radial dikes (red dots, red rose diagram) occur in the vicinity of volcanoes with orientations related to local, magmatically perturbed stresses. (C) Distribution of volcanic cones and the orientation of dike-feeders in evolved rifts in the eastern branch. In these evolved rifts, strain focuses into magmatic segments [Keranen et al., 2004], and dike trends provide no evidence for radial diking and mechanical interactions between basin segments in potential transfer zones.
What are the tectono-magmatic controls on dike orientations?
Dike orientations in the East African Rift are typically controlled by the regional least compressive stress (σ3), which should be subparallel to plate motion vectors (Saria et al., 2014). Dikes injected into rift basins in East Africa are thus expected to exhibit N-S to NNE-SSW orientations (Figure 1). However, as shown in Figure 1, intrusions in Tanzania and the DRC diverge from this expected pattern. Based on our analysis, we infer that these complex geometries may be the result of magmatic stresses (e.g., pressurized magma chambers; Gudmundsson, 1998) and mechanical interactions between rift basin segments (e.g., Pollard & Aydin, 1984). These effects are locally imposed on the regional stress state, thereby modifying the orientation of σ3 (Figure 3). Additionally, the effects of volcanic edifice loading on dike orientations should not be completely ruled out (Le Corvec et al., 2015). Volcanoes may also produce lateral stress gradients away from the summit, allowing dikes to propagate significant distances laterally from shallow magma chambers (10s of km) (Hurwitz et al., 2009). The stress directions deduced from our analyses, in combination with previous fault kinematic studies, show that locally modified stress fields, rather than pre-existing weaknesses (e.g., Proterozoic shear zones), are the primary control on the formation of transverse structural fabrics (e.g., faults and dikes). This conclusion contrasts with previous assertions from structural studies in Kenya and Tanzania (e.g., Le Turdu et al., 1999).
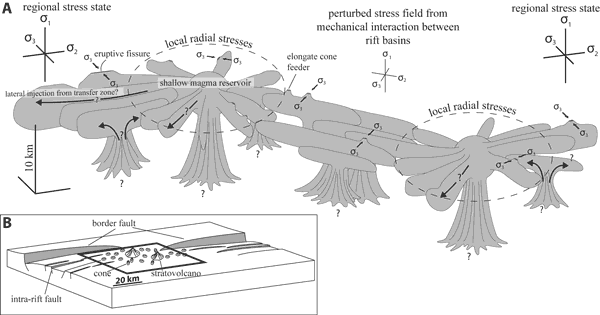
Figure 3: (A) 3-D conceptual illustration of shallow dike networks in transfer zones from Muirhead et al. (2015). The strikes of intrusions are controlled by variations in the stress state within the transfer zone. Rift-parallel dikes, with orientations controlled by the regional stress state, intrude the distal ends of the rift basins. Zones of shallow magma storage, situated below active volcanoes, create a local magmatic stress field responsible for the formation of radial dikes. These shallow magma reservoirs are assumed to be fed from below. Extension-oblique dikes form under a rotated stress field driven by the mechanical interaction between adjacent rift segments. Erupted dikes are either fed laterally from shallow magma reservoirs or ascend from mantle depths. The ascent path of magma below the upper few kilometers of the crust cannot be constrained from the data presented in this study. (B) Inset showing the structural location (transfer zone) of the volcanic cones fed by the dike network presented in A (region indicated by box). Click here or on Figure for enlargement.
What is the role of diking in early-stage rifting?
Dike-rifting events in early-stage portions (<10 Ma) of the eastern branch are rare. Although a dike intruded recently in the Natron basin of Tanzania (July, 2007), the length of this intrusion was short (<10 km) and locally confined to Oldoinyo Lengai volcano at the southern end of the basin, and in close proximity to a transfer zone. Our observations show that, in the last ~1 million years, surface-breaching dikes have rarely intruded along the central axis of early-stage rift basins (e.g., Natron and Magadi basin). This suggests that dikes play a limited role in accommodating upper crustal extension in this part of the rift. Therefore, we assert that recent observations of dike intrusions within these early-stage basins only reflect the local importance of dike-driven rifting to particular structural zones (i.e., transfer zones), rather than illustrating a basin-wide mode of upper crustal strain accommodation.
References
-
Buck, W. R. (2004), Consequences of asthenospheric variability on continental rifting, Rheology and Deformation of the Lithosphere at Continental Margins, edited by Karner, G. D., Taylor, B., Driscoll N. W. and Kohlstedt, D. L., Columbia Univ. Press, New York, 1-30.
-
Buck, W. R., L. L. Lavier, and A. N. B. Poliakov (2005), Modes of faulting at mid-ocean ridges, Nature, 434, 719-723.
-
Calais, E., et al. (2008), Strain accommodation by slow slip and dyking in a youthful continental rift, East Africa, Nature, 456(7223), 783-788.
-
Gudmundsson, A. (1998), Magma chambers modelled as cavities explain the formation of rift zone central volcanoes and their eruption and intrusion statistics, Journal of Geophysical Research, 103, 7401-7412.
-
Hurwitz, D. M., S. M. Long, and E. B. Grosfils (2009), The characteristics of magma reservoir failure beneath a volcanic edifice, Journal of Volcanology and Geothermal Research, 188, 379-394.
-
Keir, D., I. D. Bastow, G. Corti, F. Mazzarini, and T. O. Rooney (2015), The origin of along-rift variations in faulting and magmatism in the Ethiopian Rift, Tectonics, 34, 464-477.
-
Kiyosugi, K., C. B. Connor, P. H. Wetmore, B. P. Ferwerda, A. M. Germa, L. J. Connor, and A. R. Hintz (2012), Relationship between dike and volcanic conduit distribution in a highly eroded monogenetic volcanic field: San Rafael, Utah, USA, Geology, 40, 695-698.
-
Korme, T., J. Chorowicz, B. Collet, and F. F. Bonavia (1997), Volcanic vents rooted on extension fractures and their geodynamic implications in the Ethiopian Rift, Journal of Volcanology and Geothermal Research, 79, 205-222.
-
-
-
Le Turdu, C., J. P. Richert, J.-P. Xavier, R. W. Renaut, J.-J. Tiercelin, J. Rolet, K. E. Lezzar, and C. Coussement (1999), Influence of preexisting oblique discontinuities on the geometry and evolution of extensional fault patterns: evidence from the Kenya Rift using SPOT imagery in Geoscience of Rift Systems – Evolution of East Africa, edited by C. K. Morley, American Association of Petroleum Geologists, Tulsa, OK, USA, 173–191.
-
Mazzarini, F., T. O. Rooney, and I. Isola (2013), The intimate relationship between strain and magmatism: A numerical treatment of clustered monogenetic fields in the Main Ethiopian Rift, Tectonics, 32, 49-64.
-
-
Pollard, D. D., and A. Aydin (1984), Propagation and linkage of ocean ridge segments, Journal of Geophysical Research, 89, 17-28.
-
Saria, E., E. Calais, D. S. Stamps, D. Delvaux, and C. J. H. Hartnady (2014), Present-day kinematics of the East African Rift, Journal of Geophysical Research-Solid Earth, 119, 3584-3600.
-
Wright, T. J., et al. (2012), Geophysical constraints on the dynamics of spreading centres from rifting episodes on land, Nature Geoscience, 5, 242-250.
last updated 14th
August, 2015 |