 |
Venusian
Craters and the Origin of Coronae* |
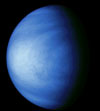 |
|
C. Vita-Finzi1,
R.
J. Howarth2, S.
Tapper3 & C.
Robinson4
1Department of Mineralogy,
Natural History Museum, London SW7 5BD, UK,
cvitafinzi@aol.com
2Department of Earth Sciences, University
College London, London WC1E 6BT, UK, r.howarth@ucl.ac.uk
3Geografx, 6 Foresters Terrace, Teignmouth,
Devon TQ14 8BP, UK, simon_tapper@btinternet.com
4Department of Remote Sensing, Boston
University, Boston MA 02215, USA, cordula@crsa.bu.edu
*This web page is an expanded version of
an abstract presented at the 35th Lunar and
Planetary Science Conference in Houston, TX,
in March 2004.
|
Introduction
Discussion of the geological
evolution of Venus generally assumes that the
planet underwent widespread, if not complete,
resurfacing by volcanism about 500 Myr ago. In
the absence of active plate tectonics - so the
argument runs - Venus is heating up [1], although
some heat is being lost from the interior by conduction
through the crust and, more importantly, through
several hundred diapiric structures which are
manifested at the surface by the circular-to-oval
structures 100 - 2600 km in diameter known as
coronae [2].
The present study suggests that
many coronae are impact craters and argues that
the wide range of morphologies displayed by the
combined population of coronae and acknowledged
craters is due to variations over time in the
nature of the target material and of the venusian
atmosphere. |
Data
Early investigators interpreted
some coronae as ancient impact craters which had
been deformed by endogenous processes or were
in some way degraded [3]; it was also suggested
[4] that coronae represented volcanism that had
been triggered by impacts. The resurfacing thesis
arose from the finding that Venus displays few
obviously recognisable impact craters, that they
are little deformed, and that their distribution
is very uniform [5]. Crater-counts gave an age
for much of the planet of 300 - 700 Myr, and the
time taken for resurfacing as 10 - 100 Myr [6].
Resurfacing has recently been questioned [7] following
reassessment of the directional model of Venusian
evolution and of the distribution of impact craters.
The corona-count of 362 in the
original survey [2] was based on Magellan imagery
covering > 90% of the planet. Altimetric data
and USGS synthetic stereoscopic images then revealed
a large number of additional coronae which were
difficult to detect on SAR imagery, largely because
they lacked a fracture annulus [8]. Addition of
the 106 new coronae gives 54% to groups 4, 6,
7 and 8 (Table 1); that is to say, over half the
coronae have the same cross-sectional form as
unaltered or flooded impact craters on the Moon
or other planetary bodies. Extending the comparison
to impact craters with central peaks by adding
coronae of group 3 would increase the proportion
to over 67%. Put another way, after allowing for
the 14% nondescript coronae of group 9 [9], only
19% of coronae would be out of a place in a catalogue
of lunar impact craters if judged by their cross-sectional
form. Even then, Gosses Bluff, in the Northern
Territory of Australia, shows that differential
erosion can invert crater morphology by selectively
removing material outside the crater rim to leave
the crater and its contents standing above the
surrounding plain in the style of corona groups
2 and 3a.
Venusian impact craters are usually
identified by having sharp rims bordered by asymmetrical
radar - bright ejecta blankets, whereas typical
coronae lack both (Figure 1). The larger impact
craters, such as Mead or Cleopatra (d = 100 km),
lack central peaks and, like their lunar counterparts,
have multiple rims. Many comparable coronae of
size range d ~ 125 -870 km [2] display a concentric
double ring. The survival of ejecta blankets is
of course a matter of the prevailing erosional
regime: no subaerial crater on Earth has yet been
found to display such a flamboyant feature, even
when allowance is made for the highlighting produced
by SAR imaging. This is presumably because weathering
and erosion would soon obscure any such feature.
Some ejecta degradation by weathering and wind
has been detected on Venus. In any case, the observation
that impact craters are all in pristine condition
[10] is self - fulfilling, because degraded craters
are then discounted as potential impact features.
Most impact craters have been surrounded and partially
filled by postimpact lavas [11]. This has had
the effect of reducing their apparent depth, creating
dark floors, and partially burying any ejecta.
Many coronae are likewise associated with volcanicity,
both in their interiors and on their flanks [2].
As on Earth, departures from circularity which
are not due to embayment or breaching by flows
could reflect faulting, jointing, and other planes
of weakness.
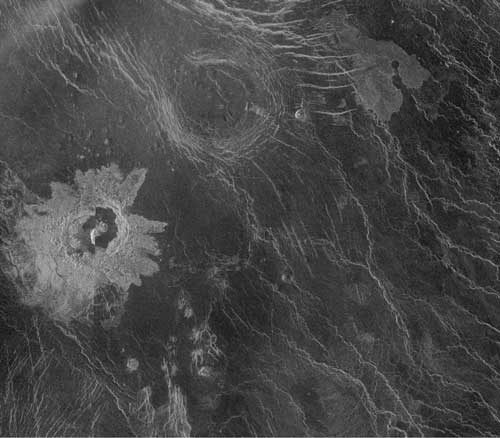
Fig. 1 Impact crater (lower
left) and corona (top centre) on Venus. ( Courtesy
of NASA) |
Statistics
An early version of this paper
[12] suggested that, apart from a few very large
features which could be genuine diapiric structures,
both impact craters and coronae fell on a lognormal
curve consistent with a single impact population
(Figure 2). A lognormal frequency distribution
is in fact a very good model for the distribution
of corona types. A two-sided Kolmogorov-Smirnov
test [13] confirms that there is no statistically
significant difference between the cumulative
distributions for the Type 1 and Type 2 coronae
(n1 =407, n2 =107, Dn =0.055; this is very much
smaller than the critical value of the Kolmogorov-Smirnov
statistic at the 0. 01 level of significance,
D84. 72, 0. 01 = 0.19; the p-value for the maximum
observed difference, Dn =0.055, is greater than
0.99). Although the median area of the population
of impacts is much smaller than that of the coronae,
the spread of the lognormal distributions of coronal
and impact areas is very similar.
Table 1: Classification
of coronae (after ref 9) |
|
|
1 |
Domes |
2 |
Plateaus |
3a |
rimmed plateaus |
3b |
rims with central high |
4 |
Rimmed depressions |
5 |
Outer rise, trough, inner rise |
6 |
Outer rise, trough, inner low |
7 |
Rim only |
8 |
Depressions |
9 |
No apparent signature |
|
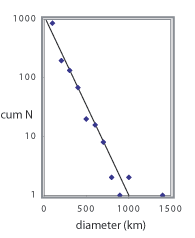
Figure 2 Plot of corona/crater diameter
in 100 km bins vs log cumulative number.
Outlier (lower right) may represent diapiric
structure. (After Vita-Finzi 1998).
|
Coronae are randomly distributed
in the plains and display strong clustering elsewhere
[2], notably in middle elevations and near chasmata
or rifts [14]. Impact craters are randomly distributed
throughout the planet, albeit with the slight
clustering inevitable with stochastic processes
[5], but, if the two populations are combined,
few areas are blank, clustering is blurred, and
there is no obvious correlation of numbers with
altitude. Although the distribution of the coronae
with latitude reflects a spatial clumping, similar
apparent weak agglomeration occurs for 1562 lunar
impacts.
Like other processes [7], corona
formation is not confined to any particular phase
in the planet's evolution. There is no correlation
between stratigraphic age, insofar as this can
be identified from the available imagery, and
corona morphology [8]. It is of course not always
possible to distinguish between the age of a rock
unit and the age of the proposed structure: the
Barringer Crater in Arizona is found in Cretaceous
rocks. However, a detailed analysis of the Scarpellini
(V33) quadrangle of the V Map at 1/5M [8] showed
that 62% of all the coronae in that quadrangle
were found on the youngest materials (“regional
plains”) and only 3% on tessera. The last
is probably an underestimate because, whether
or not tessera terrain is generally ancient, it
is characterized by intense deformation including
episodes of extension and compression, but the
observation runs counter to any assumption that
coronae reflect long-lived deep sources. Four
of the corona types identified earlier as consistent
with an impact origin (3, 4, 6 and 7) are found
in various terrains; type 8 (basins) are concentrated
in regional and lineated (i.e. tectonised) plains
[8]. Basins are considered to represent the earliest
manifestation of plume development but could be
simple craters or complex craters which have been
buried by later material. Besides overlapping
the upper end of the size distribution of impact
craters, coronae fall well within the possible
magnitudes of the rings encountered in the development
of multi - ring basins on Mars, Mercury and the
Moon, which are generally agreed to be of impact
origin.
The effect of an impact owes
much to its timing and to the atmospheric history
of the target. There is good isotopic evidence
for the equivalent in water to 0.12% of a full
terrestrial ocean formerly on Venus [15], and
some of the canali of Venus may have been cut
by running water [16]. A plausible explanation
for the loss of the water is early outgassing
“during the first billion years and associated
with intensive impacts” [17]. Some early
craters may thus have formed in regolith containing
moisture, and, as near-surface temperatures were
lower than the present ~740 K, the basalts that
dominate the Venusian surface would have been
somewhat less ductile and more retentive of small
craters. Repeated climate changes, to which small-strain
deformation features have been ascribed [18],
would doubtless complicate crater morphologies.
At the very least, earlier times will have been
characterised by a thinner atmosphere; the runaway
greenhouse that is widely held responsible for
the buildup of CO2 was cumulative, even if rapid,
and thus initially ineffective; smaller impactors
would have found it easier to reach the planet's
surface than under current circumstances. It follows
that parts of the Venusian surface are much older
than generally accepted and, consequently, that
they embody a long history of geological and climatic
change. Mismatches between the topography and
the measurable geophysical properties of Venus
would seem inevitable.
The key implication is that Venus
did not undergo resurfacing ~ 500 Myr ago and,
in the absence of plate tectonics, that it now
loses heat primarily by conduction through the
crust and along rifts. The coroane are impact
structures, and do not result from plumes or hot
diapirs. In short, the venusian greenhouse has
underfloor heating. |
References
[1] Turcotte, D. L. (1993) JGR, 98,
17061-17068.
[2] Stofan, E. R. et al. (1992) JGR,
97, 13347-13378.
[3] Greeley, R. (1987) Planetary Landscapes,
2nd ed., Allen & Unwin, London.
[4] Stewart, C. A. et al. (1993) EOS, Trans
AGU, Suppl., 26 , 80.
[5] Strom, R. G. et al. (1994) GRL, 99,
1899-10926.
[6] Phillips, R. J. et al. JGR, 97,
15923-15948.
[7] Guest, J. E. & Stofan E. R. (1998) Icarus,
139 , 55-66.
[8] Tapper, S. W. (1998) A Global Survey and
Regional Scale Study of Coronae on Venus.
PhD Dissertation, Univ. London, UK.
[9] Stofan, E. R. et al. (2001) GRL,
28, 4267 - 4270.
[10] Schaber, G. G. et al. (1992) JGR,
97, 13257-13301.
[11] Herrick, R. R. & Sharpton V. L. (2000)
JGR, 105, 20245-20262.
[12] Vita-Finzi, C. (1998) Unpublished MS.
[13] Harter, H. L. (1980) Amer. Stat.,
34 , 110 - 111.
[14] Phillips, R. J. & Hansen, V. L. (1994)
Ann. Rev. Earth. Planet. Sci., 22,
597 - 654.
[15] Donahue, T. M. et al. (1997) pp. 385-414
in Venus II, Univ. Arizona Press, Tucson.
[16] Jones, A. P. & Pickering, K. T. (2003),
J. Geol. Soc., 160, 319-327.
[17] Volkov, V. P. et al. (1986), pp. 136-187
in Chemistry and Physics of Terrestrial Planets,
Springer-Verlag, New York.
[18] Anderson, F. S. & Smrekar, S. E. (1999)
JGR, 104, 30743-30756. |
last updated September
22nd, 2004 |
|
|
|