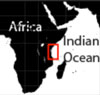 |
Intraplate deformation of oceanic crust in the West Somali Basin |
Daniel Sauter1, Jean Claude Ringenbach2, Mathilde Cannat3, Thomas Maurin2, Gianreto Manatschal1, Ken G. McDermott4
1Institut de Physique du Globe de Strasbourg, CNRS UMR 7516, 1 rue Blessig 67084 Strasbourg cedex, France, daniel.sauter@unistra.fr ; manat@unistra.fr
2TOTAL SA, CSTJF, Avenue Larribau, 64018 Pau Cedex, France, jean-claude.ringenbach@total.com ; thomas.maurin@total.com
3Institut de Physique du Globe de Paris, CNRS UMR 7154, 1, rue Jussieu 75238 Paris Cedex 05, France, cannat@ipgp.fr
4ION 31 Windsor St., Chertsey, KT16 8AT, UK, Ken.McDermott@iongeo.com
This webpage is a summary of: Sauter, D., J. C. Ringenbach, M. Cannat, T. Maurin, G. Manatschal, and K. G. McDermott (2018), Intraplate Deformation of Oceanic Crust in the West Somali Basin: Insights From Long-offset Reflection Seismic Data, Tectonics, 37, 588-603, doi: 10.1002/2017tc004700
In the West Somali Basin (WSB) the Davie Ridge (Figure 1) and its NS-trending northern extension were long thought to be the outer limit of the thinned continental domain and the Tanzanian margin seen as a ‘transform margin’. The Davie Ridge itself is inferred to be the bathymetric expression of the transform fault that accommodated the southward motion of Madagascar relative to Africa (Scrutton, 1978). Therefore, numerous deep seismic reflection profiles were shot west of the Davie Ridge, in the westernmost part of the WSB, looking for new offshore oil and gas discoveries. However, unexpectedly, some of these profiles revealed oceanic crust between the Sea Gap Ridge and the Davie Ridge and its northern extension (Figure 1). The release of some of these high-quality seismic reflection profiles was the starting point of the academic research reported here.
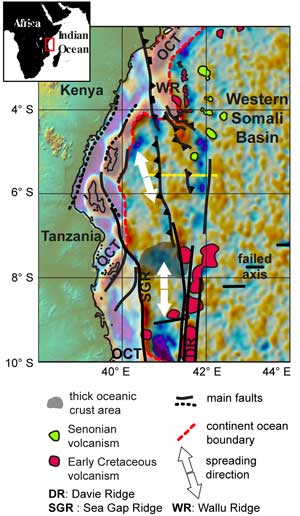
Figure 1: Structural map of the westernmost part of the West Somali Basin modified after Sauter et al. (2018). The background image is the 2' gravity anomaly grid downward continued onto the seafloor of Whittaker et al. (2008), based on Sandwell & Smith (2005). A strike-slip component is inferred along faults indicated by black lines without triangles or tick marks. Tertiary faults related to the East African rift system are not shown. The yellow line indicates the location of the seismic profile shown in Figure 2.
With a set of six new papers, the WSB has been in the spotlight for the last two years (Davis et al., 2016; Phethean et al., 2016; Reeves, 2017; Reeves et al., 2016; Sauter et al., 2018; 2016). After 40 years of debate it seems that there is now an agreement on the age of the cessation of spreading (around M0 time, Aptian, Early Cretaceous) and on the location of the extinct spreading axis. However, because of a lack of magnetic anomaly profiles close to the East African margin, the age of the first oceanic crust is still poorly constrained. The oldest magnetic anomalies identified in the WSB are M22-M24 (150-155 Ma – Late Jurassic) (Davis et al., 2016) but spreading may have begun earlier, at 170-160 Ma (e.g., Geiger et al., 2004). Spreading was directed N-S before it ceased, but a NNW-SSE direction is inferred from gravity lineaments close to the margin (Figure 1) (Davis et al., 2016; Phethean et al., 2016) .
The reflection seismic profiles presented in these new papers were acquired by ION as part of their East AfricaSPANTM program. In most of these seismic sections we found an oceanic Moho at ~2 s two-way travel-time (TWTT) below a highly reflective top basement. This led us to propose oceanic crust west of 41°30’E (Figure 1) in agreement with recent work (Phethean et al., 2016; Reeves et al., 2016; Sauter et al., 2016) . However, we observed some unusual features at the top the oceanic crust as well as below the oceanic Moho.
A series of alternating strong and weak reflectors was often observed in the upper part of the crust giving it a layered aspect. This volcano-sedimentary sequence was mostly observed within tilted fault blocks where it locally forms wedges indicating a syn-tectonic origin. The sequence thickens southward concomitantly with a reduction in crustal thickness toward the inferred fossil ridge axis. This suggests that it formed as a response to discontinuous magmatism due to the decreasing melt budget as seafloor spreading came to an end. At the extinct mid-Labrador spreading center, several observations also favor tectonic thinning of the crust when spreading approached failure (Delescluse et al., 2015).
In regions of thicker crust (Figure 1) and smoother volcanic top basement, the volcano-sedimentary sequence is not developed. This may have resulted from a magmatically robust seafloor spreading event with continuous volcanic feeding of the upper crust and without faulting. The oceanic Moho is especially clear in these thickest crustal areas where the top basement is smooth and unfaulted. There, a group of reflectors was also observed beneath the Moho and interpreted as a crust-mantle transition zone made of trapped melt bodies within the mantle (see Sauter et al., 2016)). This interpretation implies inefficient extraction of melt from the mantle.
Both the top basement and Moho show folds with a 20-30 km wavelength in the westernmost part of the WSB (Figure 2). This deformation is more severe to the north where folds are of larger amplitude than to the south and associated with large thrust faults (Figure 2). These thrusts have high-angle dips and cut across the basement to the Moho. In the upper part of the crust, the reflectors of the volcano-sedimentary sequence are usually parallel to the folded top basement indicating that the sequence was folded with the basement. Locally, some tilted blocks bounded by normal faults have been reactivated. Buckling of the crust and associated reverse faulting thus occurred after the extensional phase, which is associated with the emplacement of the volcano-sedimentary sequence [**ambiguous pls re-word]. In the southern part of the study area the oldest sedimentary layers, including Hauterivian (131-134 Ma), onlap the folded top basement while in the northern part these Hauterivian layers are affected by inversion (Figure 2). The Aptian (113-126 Ma) horizon almost seals the top basement relief everywhere. We therefore conclude that the deformation of the oceanic crust occurred soon after its formation, as early as pre-Hauterivian time, and ended in Aptian time.
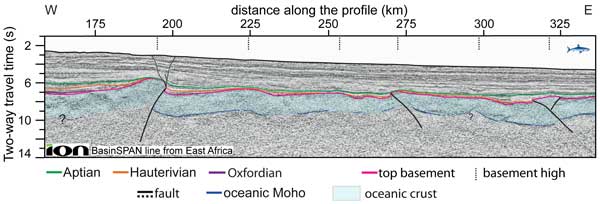
Figure 2: Seismic reflection profile with superimposed interpretation. Dashed black lines: inferred fault traces, black continuous lines: more certain fault traces. No vertical exaggeration. See Figure 1 for location. Courtesy of ION Geophysical.
Deformation is localized along corridors trending N-S to NE-SW (Figure 1) parallel to former spreading directions. Inherited segmentation of the oceanic crust due to transform faults or non-transform offsets at the ridge axis may have thus strongly influenced the deformation of the oceanic crust. Volcanic edifices corresponding to additions on top of the oceanic crust lie along the Davie Ridge and its northern extension. At depth a group of reflectors within the mantle reaches the base of these post-spreading volcanic edifices. The geometry of this set of reflectors suggests strong tectonic control of the melt channeling with faults being utilized (potentially reactivated) within the deformation corridors.
Folding and reactivation of the oceanic fracture zones ended at Aptian times when spreading ceased in the WSB. This timing of the folding and reverse faulting in the westernmost WSB is close to one of the convergence phases proposed by Gaina et al. (2015) in the North Somali Basin. Early Cretaceous transpressive structures were also inferred to the west of the Davie Ridge in the northern Mozambique Basin (Franke et al., 2015; Mahanjane, 2014). The change in plate tectonic regime off the coast of East Africa leading to this so-far overlooked Early Cretaceous convergent phase remains to be investigated.
We concluded that intraplate deformation occurred in the WSB for two reasons. First, the oceanic lithosphere was young and therefore thin enough to be folded. Second, the regional stress changed and compression became oriented oblique to the major and minor fracture zones, reactivating them and initiating thrusting and buckling.
Acknowledgements
We thank ION for permitting publication of their East AfricaSPANTM seismic data.
References
- Davis, J.K., Lawver, L.A., Norton, I.O., and Gahagan, L.M., 2016, New Somali Basin Magnetic Anomalies and a Plate Model for the Early Indian Ocean: Gondwana Research, 34, 16-28.
- Delescluse, M., Funck, T., Dehler, S.A., Louden, K.E., and Watremez, L., 2015, The oceanic crustal structure at the extinct, slow to ultra-slow Labrador Sea spreading center: J. Geophysical Research: Solid Earth, 120, 5249–5272.
- Franke, D., Jokat, W., Ladage, S., Stollhofen, H., Klimke, J., Lutz, R., Mahanjane, E.S., Ehrhardt, A., and Schreckenberger, B., 2015, The offshore East African Rift System: Structural framework at the toe of a juvenile rift: Tectonics, 34, 2086-2104.
- Gaina, C., van Hinsbergen, D.J.J., and Spakman, W., 2015, Tectonic interactions between India and Arabia since the Jurassic reconstructed from marine geophysics, ophiolite geology, and seismic tomography: Tectonics, 34, 875-906.
- Geiger, M., Clark, D.N., and Mette, W., 2004, Reappraisal of the timing of the breakup of Gondwana based on sedimentological and seismic evidence from the Morondava Basin, Madagascar: J. African Earth Sciences, 38, 363-381.
- Mahanjane, E.S., 2014, The Davie Fracture Zone and adjacent basins in the offshore Mozambique Margin – A new insights for the hydrocarbon potential: Marine and Petroleum Geology.
- Phethean, J.J.J., Kalnins, L.M., van Hunen, J., Biffi, P.G., Davies, R.J., and McCaffrey, K.J.W., 2016, Madagascar's escape from Africa: A high-resolution plate reconstruction for the Western Somali Basin and implications for supercontinent dispersal: Geochemistry, Geophysics, Geosystems, 17, 5036-5055.
- Reeves, C.V., 2017, The development of the East African margin during Jurassic and Lower Cretaceous times: a perspective from global tectonics: Petroleum Geoscience, 24, 41-56.
- Reeves, C.V., Teasdale, J.P., and Mahanjane, E.S., 2016, Insight into the Eastern Margin of Africa from a new tectonic model of the Indian Ocean, in Nemcok, M., Rybár, S., Sinha, S.T., Hermeston, S.A., and Ledvényiová, L., eds., Transform Margins: Development, Controls and Petroleum Systems, London, Geological Society Special Publications, 431, 299-322.
- Sandwell, D.T., and Smith, W.H.F., 2005, Retracking ERS-1 altimeter waveforms for optimal gravity field recovery: Geophysical Journal International, 163, 79-89.
- Sauter, D., J. C. Ringenbach, M. Cannat, T. Maurin, G. Manatschal, and K. G. McDermott (2018), Intraplate Deformation of Oceanic Crust in the West Somali Basin: Insights From Long-offset Reflection Seismic Data, Tectonics, 37, 588-603, doi: 10.1002/2017tc004700
- Sauter, D., Unternehr, P., Manatschal, G., Tugend, J., Cannat, M., Le Quellec, P., Kusznir, N., Munschy, M., Leroy, S., Mercier de Lepinay, J., Granath, J.W., and Horn, B.W., 2016, Evidence for magma entrapment below oceanic crust from deep seismic reflections in the Western Somali Basin: Geology, 44, 407-410.
- Scrutton, R.A., 1978, Davie fracture zone and the movement of Madagascar: Earth and Planetary Science Letters, 39, 84-88.
- Whittaker, J.M., Muller, R.D., Roest, W.R., Wessel, P., and Smith, W.H.F., 2008, How supercontinents and superoceans affect seafloor roughness: Nature, 456, 938-941.
last updated 5th April, 2018 |