Ercan
Aldanmaz
Department of Geology,
University of Kocaeli, Izmit 41040, Turkey
ercana@kocaeli.edu.tr
Click here to
download a PDF version of this webpage
Abstract
Western Turkey contains
a number of intra-continental alkaline volcanic eruption
sequences along localized extensional basins that developed
in relation to Late Cenozoic extensional processes.
The volcanic suites are small-volume alkaline olivine
basalts and basanites with compositions representative
of mantle-derived primary (or near-primary) melts. The
rocks have near-uniform radiogenic isotopic ratios (87Sr/86Sr=
0.70299-70354 and 143Nd/144Nd=
0.51281-0.51301) and are characterized by OIB-type trace-element
patterns with significant enrichment in LILE, HFSE and
L-MREE, and slight depletion in HREE, relative to N-MORB.
Systematic changes in melt chemistry with time do not
reflect fractional crystallization nor can they be explained
by variable proportions of mixing between melts produced
by different degrees of partial melting of two (or more)
compositionally distinct sources in the mantle. Instead,
the observed trends are consistent with a progressive
decrease in degree of melting and systematic mixing
between increments of melt derived from the same source
but probably at different depths. Incompatible-trace-element
modeling of fractionation-corrected data indicates that
mafic alkaline magmas formed by variable degrees of
incremental partial melting of a single, convective
mantle domain that is enriched in all incompatible elements
(e.g. LILE, HFSE and L-MREE) relative to compositions
of hypothetical geochemical reservoirs such as Depleted
MORB Mantle (DMM) or Primitive Mantle (PM). The calculations,
based on the melt products of experimental phase equilibria,
show that the compositions of the primary magmas precipitating
the most magnesian olivine phenocrysts of the alkaline
melts reveal anhydrous liquidus temperatures that range
from about 1320°C to 1410°C. Projection along
the solid-state adiabat to one atmosphere reveals a
mantle potential temperature Tp ~1300°C which is
inconsistent with melt generation by either deep-seated
thermal anomalies associated with mantle plumes (which
require Tp >1450°C) or by melting of thermally
perturbed (wet) mantle lithosphere, but are consistent
with the adiabatic upwelling of normal-temperature mantle
asthenosphere.
Introduction
Mantle melting linked
to either actively upwelling plumes or convectively
upwelling hot mantle materials is widely believed to
be responsible for the genesis of the considerable volume
of primary basaltic magma that constitutes the mafic
volcanic suites of oceanic islands and intra-continental
extensional settings (e.g., McKenzie &
Bickle, 1988). Many recent papers have alternatively
linked the origin of small volumes of primary melt to
direct melting of thermally perturbed mantle lithosphere.
Models of melt generation from chemically distinct parts
of mantle domains have been developed (Meibom
& Anderson,
2003; Foulger
& Anderson,
2005; Foulger
et al.,
2005). The primary melt products of adiabatic decompression
melting in the upper mantle preserve information on
the composition of the mantle and the mechanisms and
extents of melt extraction.
In western Turkey,
Late Miocene to Quaternary volcanic activity (11 to
0.13 Ma; Aldanmaz, 2002; Aldanmaz
et al.,
2006) produced a series of scattered outcrops of
silica-undersaturated, mafic alkaline lava flows along
localized extensional zones (Figure 1). Many recent
studies have suggested that this alkaline magmatism
is related to the late-stage extension that occurred
as a consequence of post-collisional strike-slip and
extensional faulting (e.g., Aldanmaz et al.,
2000 and references therein).
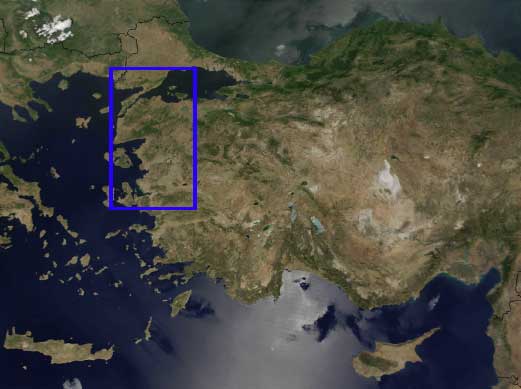
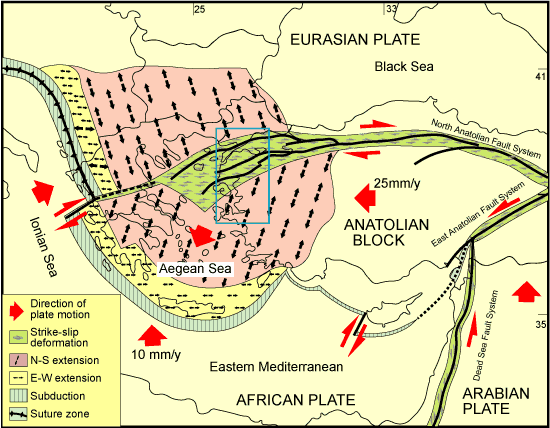
Figure 1: (a) Satellite
map of western Turkey. (b) Map showing the plate reconstruction
of the eastern Mediterranean and the area of volcanism
in western Turkey (shaded).
In recent years,
attempts have been made to constrain the melt generation
processes that produce the alkaline magmas of western
Turkey. Some researchers have proposed mixing of melts
from discrete mantle components, a model which is invoked
to explain the formation of many OIB-type (both oceanic
and continental) alkaline suites elsewhere. These studies
mainly used trace-element and isotopic data to propose
that the extensional mafic volcanic rocks of western
Turkey were derived from melts generated within mantle
lithosphere that had previously been enriched by melt
fractions from the mantle asthenosphere (e.g., McKenzie
& O’Nions, 1995; Alici et al.,
2002; Editor's note: see also The
Metasomatic Alternative). However, other studies
(e.g., Aldanmaz et al., 2000), having constrained
the likely compositions of the variable mantle regions
beneath western Turkey, demonstrated the improbability
of mantle lithosphere source involvement in the genesis
of the alkaline magmas. Instead, a well-mixed, homogeneous
convecting upper mantle was proposed as the main source.
This webpage presents
the geochemical characteristics of the primitive alkaline
volcanic rocks from western Turkey in order to provide
insights into the nature and characteristics of the
mantle source. Models involving contributions from lithologically
and chemically discrete mantle components are also evaluated.
Western Turkey is a good place to study the characteristics
of the source mantle because the erupted basalts are
relatively free from the complicating effects of mantle
lithosphere or crustal interactions and several lavas
have bulk compositions that may approximate primary
melts. Chemical data from these rocks may thus provide
the essential evidence to constrain the nature of the
melting and characteristics of the mantle source.
Geological
setting
Western Turkey is
an active continental extensional shear zone influenced
largely by forces related to both post-orogenic Aegean
extension and strike-slip faulting consequential to
westward motion of the Anatolian plate (Figure 1). Extension-related
tectonic and magmatic activity occurred along with lithospheric
thinning and asthenospheric upwelling (Aldanmaz
et al., 2005;
2006;
Editor's note: see also Slab
breakoff, Anatolia). Alkali basalts with primary
compositions occur at several localities and have been
used to document mantle melting episodes from the Late
Miocene to Quaternary. Compositional variation in basalts
throughout the lava sequences is limited as the rocks
are generally alkaline basalts and basanites occurring
as lava flows with OIB-type (or mid-plate) major-trace-element
and radiogenic-isotope signatures (Aldanmaz et al.,
2000; 2005).
Whole-rock
geochemistry
Major
and trace elements
The lavas comprise
a strongly alkaline series of silica-undersaturated
(SiO2= 42-50 wt.%), sodium-rich and high
magnesian (MgO= 6-14 wt.%) type. The more magnesian
samples have Mg# >0.70, are saturated with olivine
in the range Fo89-92, and could be in equilibrium
with mantle olivine. They have almost straight, sub-parallel
chondrite (CI)-normalized REE patterns with near-constant
concentration ratios and absolute REE abundances decrease
with increasing silica content through the eruptive
sequences (Figure 2a). All samples show LREE enriched
patterns on chondrite-normalized REE plots. The lavas
also have all the classic enrichments in LILE, HFSE
and L-MREE and slight depletion in HREE (e.g.,
relative to average N-MORB) that characterize OIB-type
basalts from intra-plate continental and oceanic settings
(Figure 2b). They also display prominent negative Rb,
K and Ti anomalies, which is also a common feature for
the majority of mid-plate basalts.
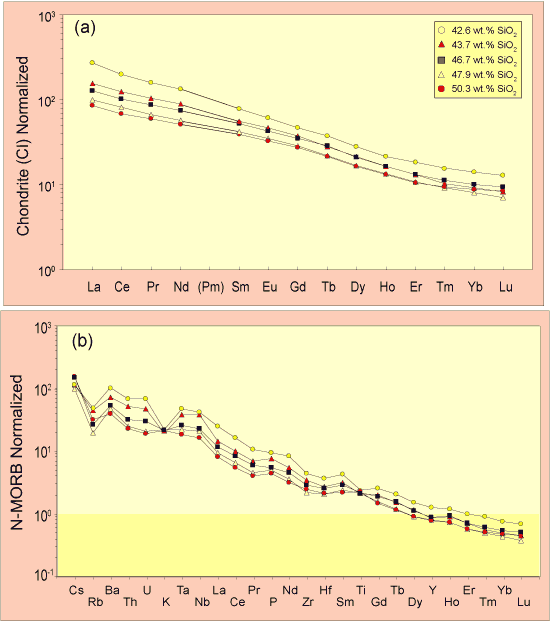
Figure 2. (a) Chondrite-
(b) N-MORB-normalized trace-element patterns for the
alkaline volcanic rocks from western Turkey. Chondrite
and N-MORB normalizing values are from Boynton (1984)
and Sun & McDonough (1989), respectively.
There are no significant
differences in trace-element ratios between the primary
and slightly evolved lavas, indicating that differentiation
played only a minor role. Incompatible-element concentrations
correlate with both silica and age; in the individual
eruptive sequences almost all incompatible-element concentrations
increase with decreasing silica contents towards the
top of the sequences. This trend cannot be explained
by fractional crystallization, as compatible major-element
concentrations such as MgO do not vary systematically
with stratigraphic height. The largely primitive compositions
of the alkaline magmas also preclude a significant role
for fractional crystallization in generating the compositional
trends. Moreover, SiO2 is negatively correlated
with incompatible elements in all sequences, the opposite
of what would be expected from fractional crystallization
of olivine and pyroxene. This overall chemical trend
is accompanied by a lithologic shift from alkali basalts
to basanites, suggesting a long-term trend of progressively
lower degrees of melting through multiple eruptive episodes.
Thus, much of the correlated variation in the major
and trace elements with stratigraphy is likely to result
from variation in the degree of melting rather than
fractional crystallization.
Isotope
ratios
The lavas are young,
with insignificant radiogenic in-growth, indicating
that their isotopic compositions represent the composition
of their sources. The rocks are characterized by low
87Sr/86Sr (0.70299-70354) and
high 143Nd/144Nd (0.51281-0.51301)
ratios, typical of what is usually seen in OIB-type
rocks from oceanic and continental settings. They have
a restricted range of isotopic ratios throughout the
lava sequences, indicating that the mantle source remained
isotopically homogeneous during the formation of the
entire suite from the Late Miocene to the Quaternary.
The rocks can therefore be considered cogenetic in a
broad sense referring to their derivation from a single,
homogeneous mantle domain. They have near-constant 87Sr/86Sr
and 143Nd/144Nd ratios for a relatively
large range in SiO2 content from 42 to 50
wt.%. This could be explained by fractional crystallization
from an isotopically homogeneous parent magma. However,
systematic change and relatively large variation in
ratios of certain trace elements, such as Zr/Nb, are
not reflected in variations in the isotopic ratios,
supporting a model of melt generation by variable degrees
of partial melting of an isotopically homogeneous mantle
source. Despite their LREE-enriched nature, the rocks
have present-day 143Nd/144Nd ratios
greater than the hypothetical bulk silicate Earth (BSE),
reflecting significant periods of evolution in LREE-depleted
reservoirs, i.e. the rocks plot within the
depleted quadrant of mantle array on a 87Sr/86Sr
vs. 143Nd/144Nd diagram, and display
significant depletions in isotopic ratios with respect
to the BSE (Figure 3a).
The statistical
distribution of 87Sr/86Sr and
143Nd/144Nd isotope data mostly
show narrow, normal distributions for both isotope ratios
(Figure 3b). This also suggests that the source mantle
from which the alkaline magmas were formed was not chemically
heterogeneous and that the contribution from lithological
outliers (e.g., a possible sub-reservoir) was
insignificant.
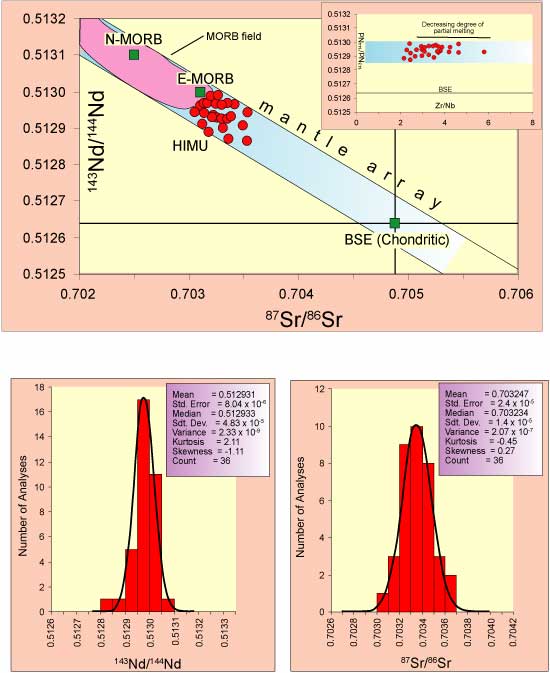
Figure 3. (a) The Nd-Sr
isotopic covariation shows that all the Late Miocene
to Quaternary mafic alkaline volcanics from western
Turkey plot in the mantle array extending from MORB-like
compositions toward BSE, and display close similarities
to HIMU. Data plotted are from Aldanmaz et al. (2000;
2006),
Alici et al. (2002), Aldanmaz (2002) and references
therein. Inset diagram shows the variation of 143Nd/144Nd
ratios with changing Zr/Nb ratios throughout the sequences.
(b) Sr and Nd isotopic distributions representing the
entire volume of the alkaline volcanic rocks, along
with the statistical parameters derived from each distribution.
Solid lines denote the fitted normal distributions.
Click here
or on Figure for enlargement.
Inferring
the nature of mantle source
Geochemistry suggests
that the alkaline lavas of western Turkey originated
from a mantle source that was enriched in all highly
and moderately incompatible elements relative to hypothetical
reservoirs such as DMM (considered to be the source
of mid-ocean ridge basalts) and PM (considered as the
source of mid-plate, OIB-type basalts) (Figure 4a-b).
The degree of enrichment in the lavas from western Turkey,
as observed in many OIB settings, increases systematically
in order of increasing incompatibility resulting in
light over heavy REE and highly-incompatible over not-so-highly
incompatible element enrichments compared to the DMM
and/or PM compositions. The isotopic results, however,
incongruently reveal an isotopically depleted (e.g.,
relative to BSE) mantle source, emphasizing that the
postulated enrichment of the lavas is a recent (possibly
process-related) event and not a long-term source characteristic.
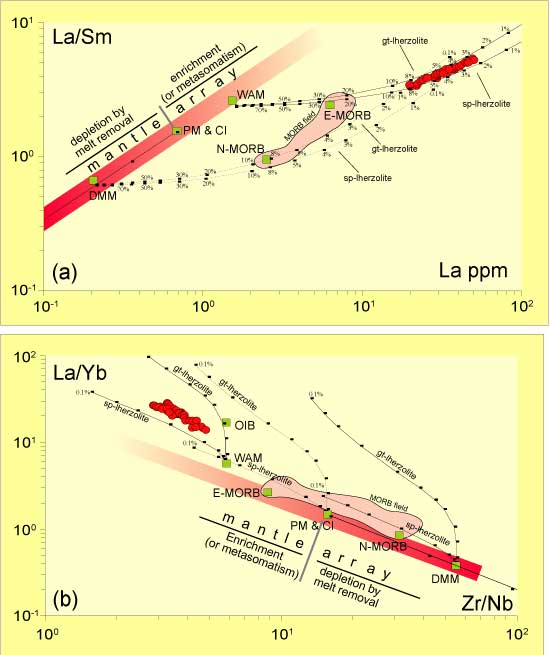
Figure 4. (a) –
(b) Plots of La vs. La/Sm and La/Yb vs. Zr/Nb showing
melting trajectories obtained using the nonmodal batch
melting. Melt curves are drawn for both spinel-lherzolite
and for garnet-lherzolite. WAM represents the Western
Anatolian Mantle defined in Aldanmaz et al. (2000).
The heavy line represents the mantle array defined using
melt-residual compositional trends from DMM and PM compositions.
Mantle depletion and enrichment trends are defined by
melt extraction from the mantle (towards the residue)
and melt addition to the mantle (or influx of material
from outside the mantle prior to solid-state mixing
and homogenization) respectively. Thick marks on each
curve correspond to degrees of partial melting for a
given mantle source. Click here
or on Figure for enlargement.
Recent enrichment,
i.e. enrichment in more-incompatible relative
to less-incompatible elements during and/or after melt
segregation from the mantle, is a common feature of
the majority of OIB lavas and could be attributed to
a number of processes, including:
- mixing of melts
from two or more chemically (or lithologically) distinct
end-members (usually requiring the involvement of
either enriched deep-plume upwellings, an enriched
mantle lithospheric source, or enriched pyroxenitic
vein components); and
- metasomatic reactions
associated with extensive porous flow (usually requiring
involvement of the lithospheric portion of the upper
mantle in melt generation). These possible options
are discussed below for the alkaline rocks of western
Turkey.
Mixing
melts from chemically distinct mantle sources and potential
end-members
Systematically
changing the proportions of mixing between melts produced
by variable degrees of partial melting of at least two
compositionally distinct sources in the mantle is often
invoked to explain both the isotopically depleted (e.g.,
relative to BSE), but LREE-enriched nature of many OIB-type
alkaline suites and the quasi-linear patterns in highly
incompatible elements observed in OIB-type rocks. Several
recent studies of trace-element and isotope compositional
variations of mid-plate basalts have suggested binary
mixing as the dominant source of chemical variation
in alkali primary suites (e.g., Class & Goldstein,
1997; Kamber & Collerson, 2000; Lassiter
et al., 2000). In this context, the most likely
hypothetical end-member reservoirs are presumed to be
located in rheologically distinct (but accessible by
surface volcanoes?) parts of the mantle, including plume
components (e.g., originating from below the
convectively stirred upper mantle), and convective and
conductive upper-mantle sources. The processes of mixing
melts from two or more compositionally distinct end-members
generally involve either the addition of incompatible-element-enriched
plume-derived melts or fluids to a depleted (e.g.,
DMM-like) mantle source prior to melt generation or,
alternatively, addition of incompatible-element-depleted
mantle-derived melts to an enriched conductive mantle
source. Thus, several recent studies regarding OIB-type
magma genesis assume that the lavas are pooled and mixed
melts and that the magma generation is related to either
a plume origin or involvement of melts from conductive
mantle or both (e.g., Lassiter et al., 2000;
Lundstrom et al., 2003).
Mantle
plume origin
The
plume hypothesis (in the sense of deep-seated thermal
anomalies), assumes (perhaps unrealistically) that the
convecting upper mantle is cold, dry and significantly
subsolidus, and generally attributes magma generation
to temperatures of approximately 250-300°C above
the ambient mantle temperature to achieve sufficient
melt for intra-plate basalt provinces (despite the volatile-rich
nature of most intra-plate sources). This hypothesis
also assumes (again, perhaps unrealistically) that the
composition of the DMM is representative of the entire
convecting upper mantle, and invokes deeper, more enriched
mantle sources to produce melts with OIB geochemical
signatures.
There
are, however, numerous problems reconciling the observations
with a plume model in the OIB-type alkaline suite of
western Turkey. Extensional stress associated with lithospheric
uplift, for instance, should be at a maximum above a
plume apex, which in turn would be expected to result
in uplift and doming. This is, however, not observed
in the alkaline field of western Turkey, nor is there
any evidence for locally elevated temperatures. In fact,
the evidence from surface heat flow measurements and
mineral equilibrium constraints (Aldanmaz
et al.,
2005) suggests that the thermal gradient in western
Turkey is not anomalously high. The bulk chemistry of
undifferentiated (or fractionation corrected) alkaline
magma compositions from western Turkey provides evidence
for “primary melts” with 11-16 wt% MgO and
liquidus temperatures ranging from 1290°C to 1410°C
(see Aldanmaz
et al.,
2005 for details). Combining these results with
information on the depth of melt extraction (Figure
5) implies that the mantle region which melted to produce
the alkaline primary compositions has a potential temperature
of ~1300°C, a value significantly lower than suggested
to be associated with mantle plumes (>1450°C;
McKenzie & Bickle, 1988). It is therefore
difficult to attribute mantle melting in this region
to plume-related thermal anomalies. In addition, the
radial thermal and stress perturbations expected from
an adiabatically upwelling mantle plume are inconsistent
with the linear distributions of the volcanic centers
as well as the planar geometry of normal and strike-slip
faulting in western Turkey, to which the small volume
and episodic alkaline magma generation is mostly related
(e.g., Aldanmaz et al., 2005;
2006).
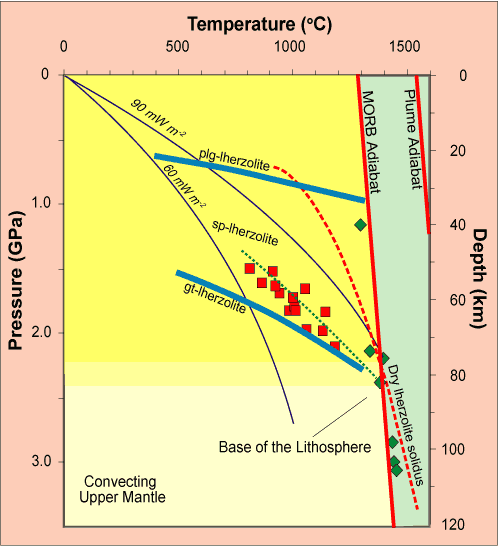
Figure 5. Thermal structure
of the northern part of western Turkey inferred from
mantle-derived ultramafic nodules and alkali primary
melts. Squares represent coarse, shallow xenoliths along
which the inferred (and laterally averaged) regional
geotherm was determined (dashed line). Closed diamonds
represent alkaline primary melts along which the inferred
ambient mantle geotherm was determined. The MORB adiabat
and the plume adiabat have potential temperatures (Tp)
of 1280°C
and 1540°C
respectively. Dry peridotite solidus shown as dashed
curve are from Herzberg et al. (2000). Conductive Continental
Geotherm curves are from Pollack & Chapman (1977).
Furthermore,
for the case of western Turkish alkaline suites there
is no clear indication of a change in depth, i.e.
from garnet- to spinel-facies mantle, as might be expected
for an actively upwelling mantle plume. Nor is there
any dramatic change in the degree of partial melting,
also expected for a mantle plume, that would potentially
include large degrees of partial melting. The alkaline
lavas from western Turkey display a trend of significant
enrichment in incompatible trace elements and a gradual
decrease in silica content with decreasing eruption
age. Such a compositional trend can be interpreted in
terms of progressively increasing depth of melting coupled
with decreasing degree of melting in a compositionally
uniform source. This is inconsistent with the plume
hypothesis.
Lithospheric
mantle origin
Involvement
of melts from the conductive mantle in the genesis of
OIB-type magmas is usually considered to be a common
process owing partly to the ability of the conductive
mantle to remain isolated from mantle convection for
periods of time geologically reasonable to create the
anomalous isotopic signatures of most OIB magmas compared
to MORB. The existence of residual hydrous minerals
such as amphibole or phlogopite (evident from the classic
negative K and Rb anomalies in most OIBs) is another
reason why many researchers believe that involvement
of conductive mantle-derived melt in OIB genesis is
inevitable (e.g., Class & Goldstein, 1997).
Melt derivation from conductive mantle for the alkaline
magmas of western Turkey is unlikely however; as noted
by Aldanmaz et al. (2000), the mantle lithosphere
beneath western Turkey is subduction-modified, and any
model involving melts from such a source would produce
magmas with significant arc signatures (e.g.,
selective enrichment in fluid-soluble LILE relative
to the insoluble HFSE). However, neither the lavas of
western Turkey nor OIB-type magmas in general display
such selective enrichments. Many oceanic and continental
alkaline primary suites worldwide are characterized
by “non-selective enrichment” in both HFSE
and LILE (relative to N-MORB), supporting a source of
melt from a homogeneous, possibly convective, region.
The
prominent negative anomalies in K, Rb and Ti (both in
western Turkish lavas and OIB-type lavas) can most likely
be explained by buffering against a K-bearing (hydrous)
phase (e.g., phlogopite and/or amphibole) in
the mantle source region. Although some argue against
the possibility of long-term stability of the inferred
hydrous minerals in a steady-state convecting system
(because these phases are not particularly common primary
mantle minerals), the existence of such phases can be
attributed to the metasomatizing effects of melts during
(or shortly after) melt generation and should not necessarily
be interpreted as a long-term source characteristic.
For
the case of the alkaline rocks from western Turkey,
the presence of the inferred hydrous phases in the original,
pre-melting, solid mantle is also inconsistent with
the temporal compositional trend of the lavas because,
irrespective of the nature of the mantle region, the
time-integrated fusion of these hydrous phases would
be expected to lower the hydrous character of the magmas
as melting precedes, which is not the case here. The
most likely explanation is therefore that the inferred
hydrous minerals are not primary phases in the source
mantle and might instead be the short-lived products
of metasomatic reaction during melting and melt migration
through intergranular channels. Supporting evidence
for melt metasomatism may be that the depletion in Rb,
K and Ti correlates with both time and degree of partial
melting (but not with the isotopic ratios) and, as shown
in K/K* vs. La plots (Figure 6), the degree of depletion
increases gradually with time towards the most primitive
sample (the product of the smallest degree of melting
with the greatest liquidus temperature) implying a time-integrated
increase in the extent of source metasomatism.
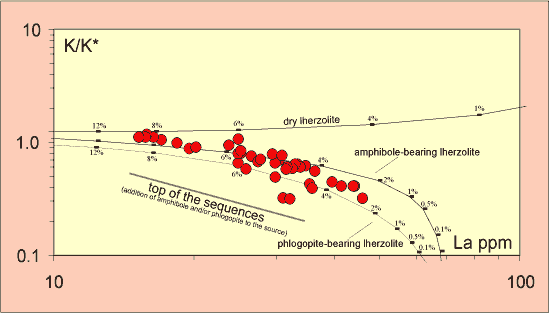
Figure 6. Covariation
of K/K* (taken as K negative anomalies) with changing
La contents of the rocks. K* has been taken as extrapolated
K calculated using normalized concentrations of Th,
K, Nb. Melt trajectories are drawn for (1) dry lherzolite
(ol0.530+opx0.270+cpx0.170+sp0.030);
(2) amphibole-bearing lherzolite (ol0.558+opx0.201+cpx0.076+sp0.115+amp0.050);
and (3) phlogopite-bearing lherzolite (ol0.558+opx0.201+cpx0.076+sp0.115+amp0.050)
sources using non-modal batch partial melting. Melting
of amphibole- and phlogopite-bearing lherzolites is
assumed to initiate in adry condition but to continue
with addition of the inferred hydrous phases by up to
5%. Click here
or on Figure for enlargement.
This
type of metasomatism can also be considered effective
in modifying the composition of the resultant melts,
because elements move through porous peridotites with
a diffusive velocity inversely proportional to their
solid/melt partition coefficients (e.g., Takazawa
et al., 1992). Under certain conditions, infiltrating
melts can be equilibrated with incompatible elements
in mantle peridotites during melt migration and ascent,
preferentially leaching incompatible elements from the
mantle peridotites. This type of melt-rock interaction
produces magma batches with high ratios of highly incompatible
to less incompatible elements relative to the original
parental melt composition.
Convective
mantle origin
Incompatible-trace-element
ratios and isotopic constraints provide the most compelling
evidence against melt generation by mixing melts from
multiple source components in the genesis of western
Turkish alkaline lavas. Rocks that formed over a significant
period of time have near-constant Sr and Nd isotopic
ratios and incompatible-element-concentration ratios
for the entire suite, indicating that melt generation
probably took place under conditions of significant
chemical homogeneity, probably within a single mantle
domain. Therefore, any model proposed to account for
the composition of the alkaline magmas from western
Turkey should be able to describe melt generation within
a well-homogenized system with no contribution from
a source that was selectively enriched in mobile incompatible
elements. The best site for this homogenization, which
might possibly require pre-melting and mixing of discrete
mantle lithologies created by plate-recycling processes
or delamination would be a sub-lithospheric, convecting
(i.e. efficiently stirred, homogeneous) system
(e.g., Hofmann, 1997; van Keken et al.,
2002; Kellogg et al., 2002; Editor's note:
see also Lower
crust recycling and Gravitational
instability ).
The
inferred mantle source seems to represent a part of
the uppermost convecting system that shows remarkable
differences in terms of geochemical characteristics
compared to the MORB source which is usually considered
the sole representative of the uppermost convecting
mantle. This can most likely be explained by the existence
of self-mixed and chemically homogeneous mantle domains
(e.g., created by plate recycling and subsequent
mixing-homogenization processes) within the convectively
stirred upper mantle.
If
interpreted in a regional context, the western Turkish
alkaline lavas exhibit strong similarities in terms
of trace-element and isotopic compositions with coeval
widespread alkaline magmatism formed in very similar
geological settings throughout a magmatic belt extending
from the central east Atlantic through southern, northern
and eastern Europe to the western Mediterranean (the
so-called “European Volcanic Province” (EVP);
Wilson et al., 1995). In particular, geochemical
characteristics of the western Turkish alkaline rocks
are almost identical to the HIMU end-member of the EVP
volcanism, which is referred to in the literature as
to the lower-velocity-component (LVC; Hoernle et
al., 1995) or the European Asthenospheric Reservoir
(EAR; Wilson et al., 1995). The existence of
this component has been attributed to a number of processes
including;
-
plume
activity from lower mantle in the central Atlantic
and sublithospheric channeling throughout North
Africa and Europe (Oyarzun et al., 1997);
-
plume-like
smaller-scale diapiric upwellings from deeper sources
(Wilson et al., 1995).
However,
a deep, lower-mantle source for this component seems
unlikely because of the thermal and compositional constraints
outlined above. This component seems to be the hallmark
of mid-plate basalts that characterize a tectonic regime
dominated by plate convergence and collision followed
by later-stage extension (i.e. the convergence zone
between the European and African plates). It is thus
likely to represent compositional, rather than thermal,
anomaly zones within the shallow convective mantle –
partially molten, shallow fertility anomaly zones. (Editor's
note: see also Paraná
basalts).
Summary
Geochemical evidence indicates
that, on average, the alkaline lavas of western Turkey
were generated by variable degrees of partial melting
of an isotopically homogeneous, single mantle domain
enriched in incompatible elements relative to hypothetical
DMM and PM. The lavas have a temporal compositional
trend indicating a progressively decreasing degree of
melting of a compositionally uniform source with a potential
temperature of ~1300°C. This, along with the other
geochemical signatures of the lavas (e.g.,
constraints on melt contribution from the conductive
mantle), suggests that melting probably occurred within
the shallow convecting system.
The alkaline lavas have
near-constant Sr and Nd isotope ratios for the entire
suite and systematic change in incompatible trace element
compositions (and ratios) of the lavas is not reflected
in the isotopic ratios, suggesting that the temporal
compositional trends are not caused by source variations.
Instead, the trends may be a function of pressure and
degree of melting, implying that parts of the isotopically
homogeneous (and a single) source are sampled systematically
as a function of pressure and degree of melting.
Acknowledgements
I would like to thank
Gillian Foulger for her help and encouragement during
the preparation of this note, and Don Anderson for his
comments on an earlier version.
References
-
Aldanmaz,
E, Pearce, J.A., Thirlwall, M.F., Mitchell, J.G.,
2000. Petrogenetic Evolution of Late Cenozoic, Post-Collision
Volcanism in Western Anatolia, Turkey. Journal
of Volcanology and Geothermal Research 102,
67-95.
-
Aldanmaz,
E., 2002. Mantle source characteristics of alkali
basalts and basanites in an extensional intracontinental
plate setting, western Anatolia, Turkey: Implications
for multi-stage melting. International Geology
Review 44, 440-457.
-
-
Aldanmaz,
E, Koprubasi, N., Gurer, Ö.F., Kaymakci, N.,
Gourgaud, A., 2006. Geochemical constraints on the
Cenozoic, OIB-type alkaline volcanic rocks of NW
Turkey: Implications for mantle sources and melting
processes. Lithos 86,
50-76.
-
Alıcı,
P., Temel, A., Gourgaud, A., 2002. Pb-Nd-Sr isotope
and trace element geochemistry of Quaternary extension
related alkaline volcanism: a case study of Kula
region (western Anatolia, Turkey). Journal of
Volcanology and Geothermal Research 115,
487-510.
-
Boynton,
W.V., 1984. Geochemistry of the rare earth elements:
meteorite studies, in P. Henderson (Editor), Rare
Earth Element Geochemistry. Elsevier, 63-114
pp.
-
Class,
C., Goldstein, S.L., 1997. Plume-lithosphere interactions
in the ocean basins: Constraints from the source
mineralogy. Earth and Planetary Science Letters
150, 245-260.
-
-
-
Herzberg,
C., Raterron, P., Jianzhong Z., 2000. New experimental
observations on the anhydrous solidus for peridotite
KLB-1. Geochemistry Geophysics Geosystems
1, 2000GC000089, 1-15.
-
Hoernle,
K., Zhang, Y.S., Graham, D., 1995. Seismic and Geochemical
Evidence for Large-Scale Mantle Upwelling beneath
the Eastern Atlantic and Western and Central-Europe.
Nature 374, 34-39.
-
Hofmann,
A.W., 1997. Mantle geochemistry: The message from
oceanic volcanism. Nature 385,
219-229.
-
Kamber,
B.S., Collerson, K.D., 2000. Zr/Nb systematics of
ocean island basalts reassessed: The case for binary
mixing. Journal of Petrology 41,
1007-1021.
-
Kellogg,
J.B., Jacobsen, S.B., O’Connell, R.J., 2002.
Modeling the distribution of isotopic ratios in
geochemical reservoirs. Earth and Planetary
Science Letters 204, 183-202.
-
Lassiter,
J.C., Hauri, E.H., Reiners, P.W., Garcia, M.O.,
2000. Generation of Hawaiian post-erosional lavas
by melting of a mixed lherzolite/pyroxenite source.
Earth and Planetary Science Letters 178,
269-284.
-
Lundstrom,
C.C., Hoernle, K., Gill, J., 2003. U-series disequilibria
in volcanic rocks from the Canary Islands: Plume
versus lithospheric melting. Geochimica et Cosmochimica
Acta 67, 4153-4177.
-
McKenzie,
D.P., Bickle, M.J., 1988. The volume and composition
of melt generated by extension of the lithosphere.
Journal of Petrology 29,
627-679.
-
McKenzie,
D.P., O’Nions, R.K., 1995. The source regions
of Ocean Island Basalts. Journal of Petrology
36, 133-159.
-
-
Oyarzun,
R., Doblas, M., López-Ruiz, J., Cebriá,
J.M. 1997. Opening of the central Atlantic and asymmetric
mantle upwelling phenomena: Implications for long-lived
magmatism in western North Africa and Europe. Geology
25, 727-730.
-
Pollack,
H.N., Chapman, D.S., 1977. On the regional variation
of heat flow, geotherms and lithospheric thickness.
Tectonophysics 38, 279-296.
-
Sun,
S.–s., McDonough, W.F., 1989. Chemical and
isotopic systematics of oceanic basalts: implications
for mantle composition and processes. In: Saunders
A. D., Norry M. J. (Eds.), Magmatism in the
Ocean Basins. Geological Society of London,
Special Publication 42, 313-345.
-
Takazawa,
E., Fred, F.A., Shimizu, N., Obata, M., Bodinier,
J.-L., 1992. Geochemical evidence for melt migration
and reaction in the upper mantle. Nature
359, 55-58.
-
van
Keken, P.E., Hauri, E.H., Ballentine, C.J., 2002.
Mantle mixing: the generation, preservation, and
destruction of chemical heterogeneity. Annual
Reviews in Earth and Planetary Sciences 30,
493-525.
-
Wilson,
M., Downes, H., Cebria, J.M., 1995. Contrasting
fractionation trends in coexisting continental alkaline
magma series; Cantal, Massif Central, France. Journal
of Petrology 36, 1729-1753.
last updated 14th
February, 2006 |