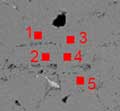 |
High water content in primitive continental flood basalts |
Qun-Ke Xia1, Yao Bi2, Pei Li1, Wei Tian3, Xun Wei4, Han-Lin Chen1
1School of Earth Sciences, Zhejiang University, Hangzhou, China; qkxia@zju.edu.cn ; peili@zju.edu.cn ; hlchen@zju.edu.cn
2School of Earth and Space Sciences, University of Science and Technology of China, Hefei, China; biyao@mail.ustc.edu.cn
3School of Earth and Space Sciences, Peking University, Beijing, China; davidtian@pku.edu.cn
4Key Laboratory of Marine Geology and Environment, Institute of Oceanology, Chinese Academy of Sciences, Qingdao, China; weixun@yeah.net
This webpage is a summary of Xia, Qun-Ke, Yao Bi, Pei Li, Wei Tian, Xun Wei & Han-Lin Chen, High water content in primitive continental flood basalts, Scientific Reports 6, Article number: 25416 (2016) doi:10.1038/srep25416
Introduction
In principle, the generation of continental flood basalts (CFB) requires abnormally high temperature, extended decompression, mafic rock in the mantle source, or H2O and/or CO2 in the mantle source (Campbell, 2001; Sobolev et al., 2011). These four factors are not mutually exclusive, and it is likely that several or all factors contribute together to generate CFB. Elevated H2O and/or CO2 content allows melting to start at greater depth and enlarges the whole melting region, consequently boosting melt volume. The CO2 content is much less than the H2O content in the mantle (Marty, 2012), and CO2 lowers the solidus less than H2O (Green et al., 2015). H2O is thus expected to be more important to the genesis of CFB. High temperature, decreased pressure and mafic source lithology have been discussed for three decades (White & McKenzie, 1989; Campbell & Griffiths, 1990; Anderson, 1995, 2005; Foulger, 2002; Sobolev et al., 2011; Hole et al., 2015), but less is known about H2O.
Measuring H2O content in basaltic magma using clinopyroxene phenocrysts
Because the partition coefficient of H2O between clinopyroxene (cpx) and basaltic melt is well constrained experimentally (Hauri et al., 2006; O’Leary et al., 2010), early crystallized cpx phenocrysts can be used to infer the initial H2O content of basaltic magma (Wade et al., 2008). Recent studies have shown that if the OH bands in cpx are approximately 3640–3620, 3540–3520 and 3470–3450 cm-1 and the maximum linear absorption is <0.3, Fourier Transform Infrared Spectroscopy (FTIR) analysis with an unpolarized beam conducted on a single cpx grain can allow estimation of H2O content with a 20% deviation at most from the result obtained by polarized IR analysis along the principal optical directions of cpx. The latter method is theoretically most accurate but difficult to apply to cpx phenocrysts in basalts because of their small sizes and the presence of cracks (Xia et al., 2013; Liu et al., 2015). Up to now, this has been the easiest way of measuring H2O content in cpx phenocrysts. For most augite and diopside phenocrysts in basalts, the H2O content can be obtained with an uncertainty of <30% and that of basaltic magma with an uncertainty of <40% (Liu et al., 2015).
Results and discussion
The Tarim basin in NW China is an early Permian large igneous province (LIP) with > 200,000 km2 of flood basalts (Figure 1). Many cpx macrocrysts (1-15 mm in size) were found in a basaltic dyke that crosscuts to the Early Permian (~280 Ma) Xiaohaizi wehrlite intrusion in the western Tarim basin. They commonly have a high-Mg core (Mg# 80-89) and a thin low-Mg rim (Mg# down to 70) resulting from interaction with the host basalt (Wei et al., 2015). The preservation of the high-Mg character and depleted Sr-Nd isotope compositions (87Sr/86Sri = 0.7035-0.7037, and εNdi = 4.5-4.8) suggest that the cores of these cpx macrocrysts may have recorded the composition of the primitive Tarim basaltic melts, with little crustal contamination (Wei et al., 2015).
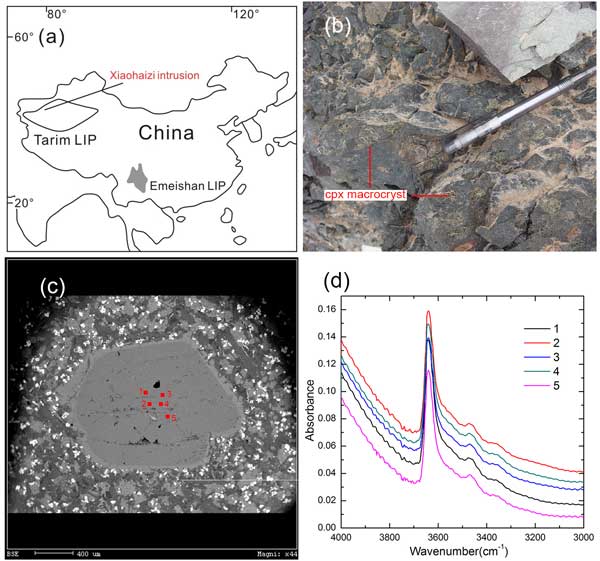
Figure 1: (a) Location of the Tarim LIP and Xiaohaizi intrusion. (b) Clinopyroxene macrocrysts in the Xiaohaizi dyke. (c) Backscattered electron image of a typical clinopyroxene macrocryst with a gray core and a thin, bright rim. Red squares are five spots used for FTIR and EPMA analyses, and the inserted figure shows the IR spectra of five spots that are the same both in band position and height.
The chemical composition and H2O content in 10 cpx macrocrysts were obtained by an electron probe micro-analyzer (EPMA) and an FTIR. Only the core of each grain was measured in order to retrieve information about the initial and primitive basaltic melts. Five to seven clean analyzed spots in the core area were selected to run EPMA and FTIR for each grain. In individual grains they show the same chemical compositions and IR spectra (Figure 1). The average values of the analyzed spots of each grain were thus used to represent the element and H2O contents of that grain. The cpx Mg# values are 85.2 to 87.8, corresponding to a Mg# of ~70 for the equilibrated basaltic melts using the experimental Mg-Fe partition coefficient (0.34 ± 0.04; Kinzler, 1997). This suggests that the analyzed cpx grains crystallized from a nearly primary basaltic source (Frey et al., 1978), in agreement with the trace-element and Sr-Nd isotope characteristics.
The H2O contents of 10 cpx grains are 300-550 wt. ppm (Average: 384 ± 83 wt. ppm), and the calculated H2O contents of the equilibrated basaltic melts are 3.69 wt.% to 6.61 wt.% (Average: 4.82 ± 1.00 wt.%) (Figure 2). Although the uncertainty is up to 40%, such an H2O content is apparently higher than those of MORB, OIB and back-arc basin basalts (0.2 - 2.0 wt.%). Instead, it falls in the range of arc magmas (2.0 - 8.0 wt.%; Figure 2).
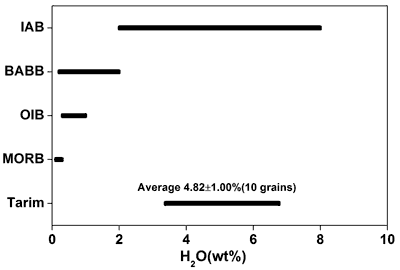
Figure 2: Comparison of the initial H2O contents of the Tarim primitive basalts with those of MORB, OIB, back-arc basin basalts (BABB) and island-arc basalts (IAB).
The arc-like H2O content of the early Permian Tarim primary basaltic melts suggests addition of water from subduction-related processes. However, the Tarim basalts do not display arc-like geochemical signatures (i.e., LILE-enrichment and HFSE-depletion; Zhou et al., 2009; Zhang et al., 2010; Yu et al., 2011; Li et al., 2012). This suggests that the extra water in the source of the Tarim basalts was not from fluids released from subducting plates but instead came from dehydrated plates stagnated in the deep Earth.
If we consider:
- the partition coefficient of H2O between mantle rock (peridotite, eclogite, pyroxenite) and melt is ~0.01 (Hauri et al., 2006), and
- the degree of partial melting of the Tarim basalts is 5-10% (Zhou et al., 2009; Li et al., 2012),
then ~2500-5000 ppm wt. H2O in the source can produce 5% H2O in basaltic melts, regardless of the melting model used (batch or fractional).
The upper mantle can accommodate several hundred ppm (wt.) of H2O (Hirschmann et al., 2005; Ohtani, 2015) and the lower mantle contains much less (Panero et al., 2015). Only the mantle transition zone (MTZ) can contain >1 wt.% H2O (Kohlstedt et al., 1996; Pearson et al., 2014). Several thousand ppm (wt.) of H2O in the source of the Tarim basalts thus probably arises from the MTZ where subducted plates stagnate and provide water (Fukao et al., 1992). If so, the classic core-mantle boundary-derived plume model (Campbell & Griffiths, 1990; Xu et al., 2014) cannot be applied to the Tarim LIP.
In conclusion, the high water content in the primary Early Permian Tarim basalts provides clear evidence that water, in addition to temperature, pressure and source lithology, plays an important role in the generation of continental flood basalts. Furthermore, when high water content is considered, abnormally high temperature and extended decompression, two critical factors in the widely accepted mantle plume model (Campbell, 2001), are not always required in the generation of CFB and LIPs.
Acknowledgements
Financial support was provided by National Natural Science Foundation of China (Nos. 41225005 and 41330207).
References
- Anderson, D. L. Lithosphere, asthenosphere, and perisphere. Rev. Geophys. 33: 125-149 (1995).
- Anderson, D. L. Large Igneous Provinces, Delamination, and Fertile Mantle. Elements 1: 271-275 (2005).
- Campbell, I. H. & Griffiths, R. W. Implications of mantle plume structure for the evolution of flood basalts. Earth Planet. Sci. Lett. 99, 79-93 (1990).
- Campbell, I. H. Identification of ancient mantle plumes. In Ernst R.E. and Buchan K.L. (eds.), Mantle Plumes: Their identification Through Time. Boulder, CO: Geological Society of America, Special Paper 352, pp. 5-21 (2001).
- Ernst, R. E. Large Igneous Provinces. Cambridge University Press. 653 pp (2014).
- Foulger, G. R. Plumes, or plate tectonic processes? Astron. Geophys. 43, 619-623 (2002).
- Frey, F. A., Green, D. H. & Roy, S. D. Integrated models of basalt petrogenesis: a study of quartz tholeiites to olivine melilitites from South Eastern Australia utilizing geochemical and experimental petrological data. J. Petrol. 19, 463-513 (1978).
- Fukao, Y., Obayashi, M., Inoue, H. & Nenbai, M. Subducting slabs stagnant in the mantle transition zone. J. Geophys. Res. 97, 4809-4822 (1992).
- Green, D. H. Experimental petrology of peridotites, including effects of water and carbon on melting in the Earth’s upper mantle. Phys. Chem. Mineral. 42, 95-122 (2015).
- Hauri, E. H., Gaetani, G. A. & Green, T. H. Partitioning of water during melting of the Earth's upper mantle at H2O-undersaturated conditions. Earth Planet. Sci. Lett. 248, 715-734 (2006).
- Hirschmann, M. M., Aubaud, C., Withers, A. C. Storage capacity of H2O in nominally anhydrous minerals in the upper mantle. Earth Planet. Sci. Lett. 236, 167-181 (2005).
- Hole, M. J. The generation of continental flood basalts by decompression melting of internally heated mantle. Geology 43, 311-314 (2015).
- Kinzler, R. J. Melting of the mantle peridotite at pressures approaching the spinel to garnet transition: application to mid-ocean ridge basalt petrogenesis. J. Geophys. Res. 120, 853-874 (1997).
- Kohlstedt, D. L., Keppler, H. & Rubie, D. C. Solubility of water in α, β and γ phases of (Mg, Fe)2SiO4. Contrib. Mineral. Petrol. 123, 345-357 (1996).
- Li, Y. Q., Li, Z. L., Sun, Y. L., Santosh, M., Langmuir, C. H., Chen, H. L., Yang, S. F., Chen, Z. X. & Xu, Y. Platinum-group elements and geochemical characteristics of the Permian continental flood basalts in the Tarim basin, northwest China: Implications for the evolution of the Tarim Large Igneous Province. Chem. Geol. 328, 278-289 (2012).
- Liu J., Xia Q. K., Deloule E., Ingrin J., Chen H., Feng M. Water content and oxygen isotopic composition of alkali basalts from the Taihang Mountains, China: recycled oceanic components in the mantle source. J. Petrol. 56(4): 681-702 (2015).
- Marty, B. The origins and concentrations of water, carbon, nitrogen and noble gases on Earth. Earth Planet. Sci. Lett. 313-314, 56-66 (2012).
- Ohtani, E. Hydrous minerals and the storage of water in the deep mantle. Chem. Geol. 418, 6-15 (2015).
- O'Leary, J. A., Gaetani, G. A. & Hauri, E H. The effect of tetrahedral Al3+ on the partitioning of water between clinopyroxene and silicate melt. Earth Planet. Sci. Lett. 297, 111-120 (2010).
- Panero, W. R., Pigott, J. S., Reaman, D. M., Kabbes, J. E. & Liu, Z. X. Dry (Mg, Fe)SiO3 perovskite in the Earth’s lower mantle. J. Geophys. Res. 120, 894-908 (2015).
- Pearson, D. G., et al. Hydrous mantle transition zone indicated by ringwoodite included within diamond. Nature 507, 221-224 (2014).
- Sobolev, S. V., et al. Linking mantle plumes, large igneous provinces and environmental catastrophes. Nature 477, 312-316 (2011).
- Wei, X., Xu, Y. G., Luo, Z. Y., Zhao, J. X. & Feng, Y. X. Composition of the Tarim mantle plume: Constraints from clinopyroxene antecrysts in the early Permian Xiaohaizi dykes, NW China. Lithos 230, 69-81 (2015).
- White, R. & McKenzie, D. Magmatism at rift zones: The generation of volcanic continental margins and flood basalts. J. Geophys. Res. 94, 7685-7729 (1989)
- Xia, Q.-K., Liu, J., Liu, S.-C., Kovács, I., Feng, M. & Dang, L. High water content in Mesozoic primitive basalts of the North China Craton and implications on the destruction of cratonic mantle lithosphere. Earth Planet. Sci. Lett. 361, 85-97 (2013).
- Xu, Y. G., Wei, X., Luo, Z. Y., Liu, H. Q. & Gao, J. The Early Permian Tarim Large Igneous Province: Main characteristics and a plume incubation model. Lithos 204, 20-35 (2014).
- Yu, X., et al. Permian flood basalts from the Tarim Basin, Northwest China: SHRIMP zircon U-Pb dating and geochemical characteristics. Gond. Res. 20, 485-497 (2011).
- Zhang, C. L., Xu, Y. G,, Li, Z. X,, Wang, H. Y. & Ye, H M. Diverse Permian magmatism in the Tarim Block, NW China: genetically linked to the Premian Tarim mantle plume? Lithos 119, 537-552 (2010).
- Zhou, M. F., Zhao, J. H., Jiang, C. Y., Gao, J. F., Wang, W. & Yang, S H. OIB-like, heterogeneous mantle sources of Permian basaltic magmatism in the western Tarim Basin, NW China: implications for a possible Permian large igneous province. Lithos 113, 583-594 (2009).
last updated 28th
September, 2016 |