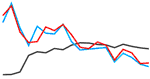 |
Are continental "adakites" derived from thickened or foundered lower crust? |
Qiang Ma1,2, Jian-Ping Zheng2, Yi-Gang Xu1, William L. Griffin3 & Rui-Sheng Zhang2
1State Key Laboratory of Isotope Geochemistry, Guangzhou Institute of Geochemistry, Chinese Academy of Sciences, Guangzhou, China, maqiang32@126.com ; yigangxu@gig.ac.cn
2State Key Laboratory of Geological Processes and Mineral Resources, China University of Geosciences, Wuhan, China, jpzheng@cug.edu.cn ; rszhang@cug.edu.cn
3ARC CoE for Core to Crust Fluid Systems/GEMOC, Macquarie University, NSW, Australia, bill.griffin@mq.edu.au
This webpage is a summary of: Ma Q., Zheng J. P., Xu Y. G., Griffin W. L., and Zhang R. S. (2015) Are continental “adakites” derived from thickened or foundered lower crust? Earth Planet. Sci. Lett., 419, 125-133, 2015. DOI: 10.1016/j.epsl.2015.02.036.
Background
The geochemical signatures of “adakites” (e.g., high SiO2, high Sr/Y and La/Yb, Nb-Ta depletion, and lack of Eu anomaly) are usually attributed to high-pressure (≥ 1.5 GPa; Defant & Drummond, 1990; Xiong et al., 2005) partial melting of mafic rocks, and accordingly the occurrence of adakitic magmas in continental settings is frequently used as an indicator for a thickened or foundered lower crust at the time of magma emplacement (e.g., Chung et al., 2003; Gao et al., 2004). These premises are built on experiments and modeling using a MORB-like source, but the probable source of continental “adakites” (i.e., continental lower crust) is compositionally different from MORB (Figure 1). To elucidate the effect of source inheritance and pressure on resultant melts, we carried out geochemical analyses and trace-element modeling on Jurassic adakitic rocks from the northern part of the North China Craton (NCC). Our study highlights the importance of source composition when defining the formation conditions of magmatic rocks in general, and in particular questions the common use of “adakites” as an indicator of specific geodynamic situations.
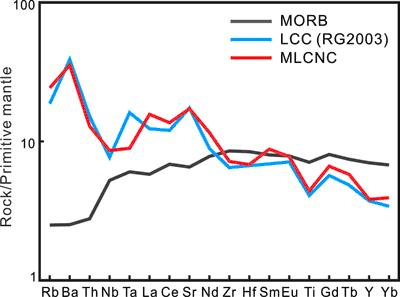
Figure 1: Primitive mantle normalized trace-element patterns of mafic lower crust of the NCC (MLCNC; this study), lower continental crust (RG2003; Rudnick & Gao, 2003) and MORB (Arevalo Jr. & McDonough, 2010).
Magma Source: Ancient Lower Continental Crust
The high-Sr/Y lavas collected range in composition from trachyandesite to rhyolite and are characterized by high Sr (>540 ppm) and LREEs, low Y (<21 ppm) and HREEs, depletion of HFSEs, a lack of obvious Eu anomalies, and low contents of MgO, Ni and Cr. In all these features they are broadly similar to the “adakite” in modern subduction zones (Defant & Drummond, 1990) and to experimental melts of mafic lower crust at 1 to1.5 GPa (Qian & Hermann, 2013). These lavas have moderate SiO2, high K2O and K2O/ Na2O (≥ 0.5), low Rb/Ba, Nb/U , Ce/Pb, and radiogenic Sr and unradiogenic Nd-Hf isotopes (model ages of ~2.5 Ga), consistent with a derivation from an ancient mafic lower crust. Such adakitic rocks are commonly considered to be products of melting of a thickened or foundered mafic LCC (Gao et al., 2004; Zhang et al., 2008). However, source composition, which is critical in the petrogenetic evaluation of igneous rocks, has not been fully considered in these models.
Origin of the Adakitic Geochemical Signature by Source Inheritance
A series of modal batch partial-melting models was designed to elucidate the effect of source inheritance and melting pressure on the origin of the adakitic rocks in the NCC. The assumed source is the mafic lower crust of the North China Craton (MLCNC), which is represented by the weighted average of Archean mafic granulite terrains and mafic lower crustal xenoliths in Phanerozoic magmas. The partition coefficients used in the calculations are from intermediate-felsic magmas and experimental runs under equilibrium conditions that would produce melting of the continental lower crust. Three different melting depths, i.e., ≤33 km, 33-40 km and >45 km, were considered, corresponding to different residual mineral assemblages defined by melting experiments (Qian & Hermann, 2013).
The results (Figure 2) suggest that melts in equilibrium with eclogitic residues (depths >45 km) from the MLCNC would be more deficient in HREE and Y, and higher in Sr/Y than the Jurassic high-Sr/Y lavas in the Yanshan belt. At lower pressures (≤40 km), with granulites or pyroxenites as residues from the MLCNC source, the modeled trace-element compositions of the melts become similar to those of the Mesozoic high-Sr/Y lavas of the NCC. For example, 5% - 30% partial melting of the MLCNC, leaving a residue without garnet or with minor garnet (<10%), produces melts that have high Sr and LREE, low Y and HREE, and thus high Sr/Y ratios. The modeling also suggests that rutile (a mineral indicator of high-pressure melting regimes) is not a necessary residual phase during the generation of the late Mesozoic high Sr/Y lavas because of the intrinsic depletion of Nb-Ta in the magma source (i.e. LCC). Moreover, high Sr concentrations and the absence of a Eu anomaly in these adakitic rocks can be attributed to source compositions and Ca-rich residual plagioclase.
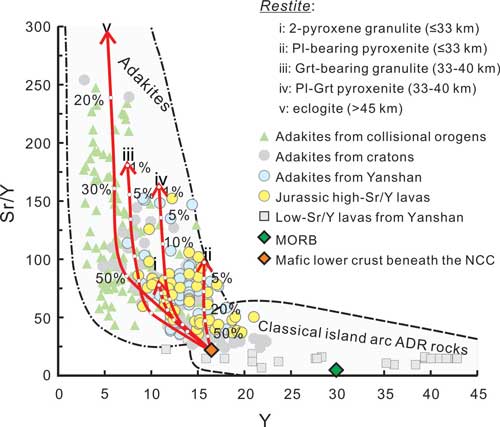
Figure 2: Plot of Sr/Y vs Y for the Mesozoic andesitic-felsic volcanic rocks in the Yanshan belt, North China. Partial-melting curves are calculated for batch melting of the mafic lower crust of the NCC (MLCNC with Y = 16.5 ppm and Sr/Y = 21) with melt fractions of 1%, 5%, 10%, 20%, 30% and 50% (white circles on each of the model curves).
Therefore, the modeling presented here strongly suggests that the main compositional characteristics of the late Mesozoic adakitic rocks in the NCC (e.g., high Sr/Y, Nb-Ta depletion, and lack of Eu anomaly) are inherited from their source rocks, rather than reflecting melting at high pressure (≥ 1.5 GPa). That is to say the continental adakitic melts in the NCC can be generated at depths of less than 40 km, and their “adakitic” signature is most likely inherited from their source rocks.
Implications for Destruction of the North China Craton
Continental adakitic rocks are widely used as a geodynamic indicator of crustal thickening, orogenic collapse and lithospheric delamination (e.g., Chung et al., 2003). This interpretation and the widespread occurrence of Mesozoic adakitic rocks in the NCC have led to suggestions of the presence of a paleo-plateau in the eastern NCC during late Mesozoic time (Zhang et al., 2008); by inference this model involves delamination of the lower crust (Gao et al., 2004). The main weakness of this hypothesis is that the effect of source composition on adakitic chemistry is not taken into account. As discussed above, the formation of such “adakitic” melts is controlled by the composition of the melting lower crust, and as a consequence, interpretations of “adakitic” rocks as markers of deep melting are far from robust. Trace-element modeling suggests that the melting depth required to produce the Mesozoic “adakitic” rocks in the Yanshan belt is from ≤33 to 40 km (Figure 2). This is similar to the thickness of the normal continental crust, which argues against a role for a thickened plateau-type crust, or its delamination, in the generation of the continental “adakites”. Other evidence supporting this argument includes analyses of paleo-drainage systems and the presence of Jurassic terrestrial fossils (plants, insects and vertebrates) that indicate a low-elevation, warm and humid environment (Jiang et al., 2008; Li et al., 2013).
Adakitic Rocks in Intraplate and Orogenic Settings
Crustal thickening rarely occurs in an intraplate setting, but may be common in orogenic settings. Deep-seated adakitic rocks (derived from >50 km depth) are therefore expected to occur in orogenic settings. We have compared intraplate and orogenic adakitic rocks in a (Sm/Yb)SN vs YbSN diagram (Figure 3). It is clear that continental “adakites” from collisional orogens generally have high (Sm/Yb)SN and low YbSN, similar to adakitic magma from modern convergent margins and to modeled melts in equilibrium with a garnet-rich residue. In contrast, continental “adakites” from ancient cratons that lack other evidence for a thickened lower crust have systematically lower (Sm/Yb)SN and higher YbSN, similar to our modeled melts in equilibrium with a residue containing little or no garnet (<10%). Consequently, the different REE budgets between adakitic rocks from cratonic areas (excluding those formed prior to cratonization) and collisional orogens can be attributed to the effects of source inheritance and high-pressure melting, respectively.
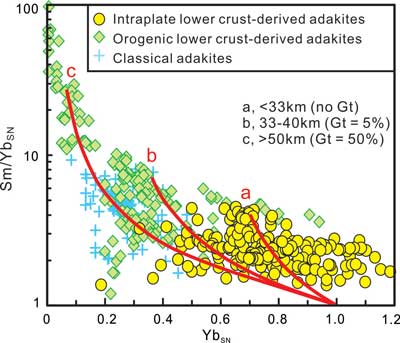
Figure 3: (Sm/Yb)SN vs YbSN diagram for adakitic rocks derived from the lower crust. The curves represent models for the partial melting of mafic rocks with resitites of (a) 2-Pyroxene Granulite without garnet, (b) Garnet-bearing Granulite with 5% garnet, and (c) Eclogite with 50% garnet. Intraplate “adakites” are represented by the Mesozoic continental adakitic rocks in the Yangtze and North China Cratons; orogenic “adakites” are from the Central Andes and continental adakitic rocks from the Tibetan Plateau and the Dabie Orogen. Subscript SN denotes source-normalized data, where the compositions of the assumed sources are MORB with Yb = 3 ppm and Sm/Yb = 1.08 for classical adakites and those from the Central Andes, and the mafic lower continental crust with Yb = 1.5 ppm and Sm/Yb = 1.87 for continental adakitic rocks.
Concluding Remarks
Variations in source composition have a profound influence on the origin of high Sr/Y “adakitic” melts in a continental setting. The case of the NCC demonstrates that the generation of “adakitic” magmas in intracontinental settings may not require a thickened or foundered lower crust. Rather they can be produced at relatively low pressures, and their peculiar compositions are inherited from their source rocks during partial melting. The role of source composition should be taken into consideration while dealing with the petrogenesis of adakitic and TTG magmas.
References
-
Arevalo Jr., R., and McDonough, W.F., 2010. Chemical variations and regional diversity observed in MORB. Chemical Geology 271, 70-85.
-
Chung, S.L., Liu, D.Y., Ji, J.Q., Chu, M.F., Lee, H.Y., Wen, D.J., Lo, C.H., Lee, T.Y., Qian, Q., and Zhang, Q., 2003. Adakites from continental collision zones: Melting of thickened lower crust beneath southern Tibet. Geology 31, 1021-1024.
-
Defant, M.J., and Drummond, M.S., 1990. Derivation of some modern arc magmas by melting of young subducted lithosphere. Nature 347, 662-665.
-
Gao, S., Rudnick, R.L., Yuan, H.L., Liu, X.M., Liu, Y.S., Xu, W.L., Ling, W.L., Ayers, J., Wang, X.C., and Wang, Q.H., 2004. Recycling lower continental crust in the North China craton. Nature 432, 892-897.
-
Jiang, H., Ferguson, D.K., Li, C., and Cheng, Y., 2008. Fossil coniferous wood from the Middle Jurassic of Liaoning Province, China. Review of Palaeobotany and Palynology 150, 37-47.
-
Li, H., Xu, Y., Liu, Y., Huang, X., and He, B., 2013. Detrital zircons reveal no Jurassic plateau in the eastern North China Craton. Gondwana Research 24, 622-634.
-
Qian, Q., and Hermann, J., 2013. Partial melting of lower crust at 10-15 kbar: constraints on adakite and TTG formation. Contributions to Mineralogy and Petrology 165, 1195-1224.
-
Rudnick, R.L., and Gao, S., 2003. Composition of the continental crust, in Rudnick R.L., ed., Treatise on geochemistry, volume 3: The crust. Oxford, UK, Elsevier-Pergamon, 1-64.
-
Xiong, X.L., Adam, J., and Green, T.H., 2005. Rutile stability and rutile/melt HFSE partitioning during partial melting of hydrous basalt: Implications for TTG genesis. Chemical Geology 218, 339-359.
-
Zhang, Q., Wang, Y., Xiong, X.L., and Li, C.D., 2008. Adakite and granite: Challenge and opportunity. Beijing, China Land Press.
last updated 8th
July, 2015 |