Why olivine control
arguments cannot be used to infer high temperature
at Samoa |
|
James
H. Natland
Rosenstiel School of Marine and
Atmospheric Science, University of Miami, Miami, FL
33149
jnatland@rsmas.miami.edu
Abstract
Olivine-liquid geothermometry
based on FeO-MgO equilibrium of picritic basalt usually
does not work because picrites almost always are
hybrid rocks, the result of mixing between differentiated
liquids on a low-temperature two- or three-phase
cotectic, and crystal sludges of more primitive magmas
rife with accumulated olivine. The mixing results
in higher FeO (here taken to be ~ 0.9 x total iron
as FeOT) in the resultant picrite than
would otherwise have been the case, producing artificially
high estimates of parental MgO, eruptive temperature,
and potential temperature from backtrack techniques.
In the case of Samoa, an additional complication
is the presence of clinopyroxene together with olivine
phenocrysts in almost every picrite; the rocks cannot
be described as “olivine-controlled”,
the essential assumption for backtrack techniques.
The influence of clinopyroxene on liquid compositions
is readily shown on simple major-oxide variation
diagrams. The effects of mixing are not so pronounced
at Vailulu`u, which is a small, active, zero-age
volcano at the eastern end of the chain with an as-yet
poorly developed flanking rift system where differentiates
would normally extensively develop. Magma is thus
more directly tapped at Vailulu`u from mantle sources,
but picrites there have the least FeO at given MgO
contents in all of Samoa, and most of them still
carry some clinopyroxene phenocrysts. This means
that they have at least some contribution from a
cotectic liquid in their makeup. Thus
no estimates of the temperature of parental liquids
in equilibrium with the Samoan mantle can yet be
made, even at Vailulu`u.
Introduction
A number of studies use FeO-MgO olivine-liquid partitioning
(e.g., Roeder & Emslie, 1970) to estimate
compositions, eruptive temperatures and potential
temperatures of primitive magmas (e.g., Larsen & Pedersen,
2000; Breddam, 2002; Herzberg & O’Hara,
2002; Putirka et al., 2007; Falloon
et al., 2007a, b; Herzberg
et al., 2007; Putirka, 2008).
The studies all identify basaltic magmas of picritic
composition, that is, with abundant olivine phenocrysts,
as the only ones liable to be descended from likely
primary magmas by means of simple olivine-controlled
differentiation beginning at elevated pressure, as
first inferred by O’Hara (1968). Since O’Hara (1968)
also said that all eruptive basalts have experienced
at least some olivine differentiation, the primary
magma is invariably a back-calculated or derived,
rather than an eruptive, composition that is presumed
to be in equilibrium with mantle olivine (~Fo91).
Of the several studies, only Falloon et al. (2007a,b)
take into account pressure in influencing FeO-MgO
partitioning (e.g., Ford et al., 1983);
this helps provide the likely range of primary compositions
at relatively low potential temperature. From experiments
in a six-component mantle-analog system Presnall & Gudfinnsson (2008)
also strongly advocate small differences in depths
and temperatures of partial melting between basalts
from islands and those from spreading ridges. All
others calculate high potential temperatures for
derived primitive compositions from islands, which
mainly have MgO contents > 20%, and this implies
convective overturn (Putirka et al., 2007, Putirka, 2008).
Convective overturn in turn supports plume models
for mantle convection.
One place outside of Hawaii that gives particularly
high potential temperatures, 223° above that
of mantle beneath spreading ridges (Purtirka
et al., 2008), is Samoa, a linear volcanic chain
in the southwestern Pacific near the Tonga Trench
(e.g., Daly, 1924; Stearns, 1944; Kear & Wood,
1959; Natland, 1980a). Background on Samoa
and a tectonic model for its origin are given in Natland (2003a).
An alternative view is given in Hart et al. (2004).
For this discussion, I take the example of Samoa
to provide a general criticism of the overall approach
taken in the olivine-melt equilibria studies, asserting
from the perspectives of petrography and bulk compositions
that no basaltic liquid so far identified in the
entire Samoan chain is truly “olivine-controlled”.
Furthermore, no
stage of simple olivine control can even be inferred
from the study of ultramafic xenoliths, many of which
crystallized from basaltic magma at depth. Instead
almost all Samoan picrites have at least clinopyroxene
together with olivine in phenocryst assemblages;
this is a typical low-pressure assemblage of alkalic
olivine basalt, producing “picritic
basalt of the ankaramite type” (Macdonald,
1949).
Data from the eastern end of the chain presented by Workman
et al. (2004) indicate that Samoan picrites are
usually mixtures between strongly differentiated magmas
with low MgO content on the one hand, and, on the other,
porphyritic, mainly olivine-rich, but also clinopyroxene-bearing, “sludges” from
high-level magma chambers that were entrained in the
differentiated magmas during their ascent and passage
through the magma conduit system. Such mixing was first
inferred for Hawaiian picrites of Kilauea volcano and
Puna Ridge ( Clague et al., 1991, 1995) and
this was later applied to picritic tuffs of the Nu`uanu
submarine landslide off of Oahu ( Natland,
2007a, b). Helz (1987) had long since provided
convincing evidence for polygenetic (mixed) origin
of olivine in Hawaiian tholeiite, including presence
of a significant proportion of deformed dunite with
subgrains – these essentially are xenoliths – and
broken, not faceted, olivine taken from crystal mats
or conduit walls. Of greatest importance is that mixing
gives the hybrid magmas unusually high FeO at a given
MgO content, but this has nothing to do with the primitive
liquid line of descent or high potential temperature.
Nevertheless, this is the erroneous basis for inferring
high potential temperatures among Samoan picrites as
well as those of other linear volcanic chains.
Picrites are Mixed Magmas
The difficulty with FeO-MgO olivine-liquid relationships
is that picrites, lavas charged with olivine phenocrysts
and which have ~12-30% MgO contents, are mixed magmas,
with olivine derived from many different primitive
mafic magma stems that mixed together (e.g.,
Natland, 1989; 2003b; 2007a,b).
A given magnesian olivine in a rock thus might not
be related to very many of the other olivine crystals
in the same rock on a liquid line of descent, nor
to the "host" liquid,
which is itself a differentiate of a hybrid primitive
mafic magma. The simplest way to test claims
of "olivine-controlled liquid" is to check
whether the rock in question is "olivine-controlled".
That is, does it only have olivine phenocrysts or
does it also have others? If it has others, then
the bulk rock is necessarily a mixture that includes
some proportion of a cotectic liquid. Then
it cannot be used for backtrack calculation, even
if some interval of olivine-only crystallization
is evident from phenocryst compositions.
This is also true even if the hybrid mesostasis,
the host glass or groundmass, is in an olivine-only
primary phase volume at eruption; mixing may have
been insufficient to saturate the hybrid with any
other phase. Indeed, the curvature of the phase boundary
may even have pulled the hybrid from the cotectic
of the multiply saturated end-member into an olivine-only
regime of crystallization (e.g., Natland,
2006). If an olivine-only episode of crystallization
occurred, then it was only in some, perhaps merely
one, of the parental liquids that contributed to
the hybrid. A single faceted euhedral clinopyroxene
crystal means that some portion of the hybrid moved
beyond this point and crystallized that clinopyroxene
on a cotectic. The crystal was in a liquid at the
time mixing occurred. Thus this clinopyroxene and
some of the melt from which it was crystallizing
inevitably comprised this mixing component. The chances
of scavenging a euhedral clinopyroxene crystal from
a crystal sludge without incorporating some of the
associated cotectic magma are extremely slim. If
it were from mantle wall rock, the crystal would
be broken, not euhedral. This likelihood makes the
hybrid glass or groundmass with a euhedral clinopyroxene
unsuitable for a backtrack calculation.
All that is necessary is look at thin sections.
Together with olivine, spinel is always there, and
electron probe microanalyses of both the spinel and
the olivine typically indicate polymagmatic sources
of both MORB and island picrites (Natland,
1989, 2003b). Otherwise, many picrites contain a
few phenocrysts of plagioclase and/or clinopyroxene
(Figure 1; e.g., from Iceland; Hansteen,
1991). In the absence of petrographic descriptions,
say when considering data files from GeoRock (e.g., Putirka
et al., 2007, Putirka,
2008), bulk-rock
compositions can be compared to those of glasses
in the same specimen, obtained by electron microprobe.
This almost always reveals that the host glasses
are low-temperature cotectic liquids – that
they experienced some degree of high-iron differentiation
involving plagioclase and clinopyroxene, with plagioclase
fractionation in particular producing high FeO.
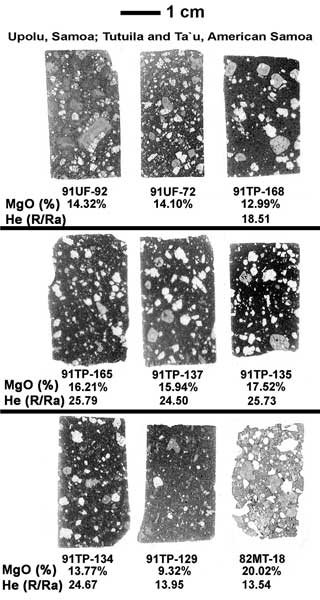
Figure 1: Scanned thin sections
of ankaramites and picrites with clinopyroxene
phenocrysts from Samoa. UF samples = those from
Fagaloa volcano, Samoa; TP samples = those from
Masefau volcano, Tutuila, American Samoa. MT sample
= one sample from Manu`a, American Samoa, Ta`u
volcano. Most samples contain olivine (light, with
cracks and irregular outlines) and clinopyroxene
(titanaugite = gray) phenocrysts. Sample 85MT-18
has two clinopyroxene phenocrysts, but the section
is too thin to show them in the scanned image.
Data for MgO contents and 3He/4He
(R/Ra) are from Farley et al. (1992). Those for
R/Ra from TP samples (Tutuila, Masefau volcanic
series) were, until recently (Jackson, 2008) the
highest known from Samoa. The sections show that
the high R/Ra of Samoan samples occurs in olivine
phencorysts of differentiated compositions.
In the Hawaiian case, the point of onset of plagioclase-clinopyroxene
cotectic differentiation occurs at about 7% MgO contents,
and corresponds to a sharp change in slope of trending
data points on many variation diagrams (FeO-MgO,
TiO2-MgO, CaO-MgO, Na2O-MgO, etc.). Of most importance,
on a diagram of MgO versus FeO, cotectic differentiation
of tholeiitic and alkalic basalt results in strong
iron-enrichment (steep negative slope), something
well known in igneous petrology for more than a century.
This follows an interval of “olivine control” at
higher MgO in which FeO does not increase. Basalt
glasses from Hawaii’s Kilauea volcano and Puna
Ridge with >7% MgO contents thus have a flatter
slope, with MgO in the glass reaching 15% (Clague
et al., 1995). Of some importance, however,
Kilauea and Puna Ridge have the only olivine-controlled
glasses known so far from anywhere in the ocean basins,
and all of them are from sand grains in a single
box core; there are no corresponding mineral analyses
for these glasses. The supposition behind all FeO-MgO
equilibria backtrack calculations is that similar
olivine-controlled glasses must exist at every other
ocean island or spreading ridge. If Hawaii has olivine-controlled
glasses, in other words, every other island chain
must have them as well. Whether they do or not, this
assumption is usually extended to the notion that
picrites generally have olivine-controlled glass
compositions. I shall show that this is far from
the case.
All glass analyses for mid-ocean-ridge basalts (MORB)
fall on a cotectic. Presnall & Gudfinnsson (2008)
emphasize the dearth of olivine-controlled glasses
on spreading ridges and Iceland, even questioning
whether picrites from Siqueiros Fracture Zone (Natland,
1989; Perfit et al., 1996) have quenched
glasses that are olivine-controlled. Falloon
et al. (2008), on the other hand, argue that
there are at least some olivine-controlled MORB glasses
including those from Siqueiros Fracture Zone.
The Siqueiros picrites cannot be discussed without
recourse to petrography, which in my view is decisive
(Natland, 2007a,b). Danyushevsky
et al. (2003) and Falloon et al.
(2007a;
2008) assert that on eruption and quenching, this
glass was crystallizing only olivine. Nevertheless,
both olivine dendrites and plagioclase spherulites
occur in the quenched margin, plus plagioclase spherulites
in embayments of olivine phenocrysts (Natland,
1980b; 1989; Perfit et al., 1996). By
analogy to programmed-cooling experiments (Kirkpatrick,
1979) the glass must be saturated in both minerals
regardless of whether olivine is the only silicate
phenocryst. Compositions of spinel, variously
included in olivine and occurring as single crystals
in glass, strongly support a mixing history among
disparate primitive magma stems in the basalt (Natland,
1989), thus neither the spinel nor the olivine enclosing
it can represent a single liquid line of descent. This
is evident in any case from data and discussion of Danhushevsky
et al. (2002). More than one picrite type
was obtained in the same dredge haul at Siqueiros
Fracture Zone, and they are not magmatically related,
even by mixing (Natland, 1989). Why
has only one of them been selected as “the” ultimate
parental MORB magma along the East Pacific Rise? Instead,
the several picrites and glass inclusions within
these and plagioclase-phyric basalts dredged nearby
likely indicate bulk lithologic heterogeneity of
the mantle within the same melting domain (Natland,
1989). Despite theoretical or experimental approaches
that are based on the assumption of a single, olivine-controlled
liquid line of descent, the relationship of the most
magnesian olivine to the host glass in the one picrite
remains uncertain.
A cotectic trend thus is easy to spot from trends
on variation diagrams, and if a picrite has a glass
composition that lies along it, this means it cannot
be used to backtrack to a parental value for MgO
contents, or to eruptive or potential temperatures
using FeO-MgO olivine-glass equilibria. The backtracked
calculated composition will necessarily have higher
FeO contents than it should for this purpose. Judging
this should be standard operating procedure before
embarking on a backtrack calculation.
I say, then, that picrites from islands and seamounts
are usually mixes between differentiated liquids on
a low-pressure, low-temperature cotectic, and one or
more sludges of magma containing a high percentage
of olivine phenocrysts ( cf., Clague et al.,
1995; Natland, 2003b; 2007a, b).
The olivine in the sludges may be so abundant because
of mechanical mechanisms of crystal sorting such as
flowage differentiation ( Drever & Johnston,
1958; Simkin,
1967; Komar, 1972, 1976; Natland,
2007a, b).
The olivine, too, usually has a differentiated composition
(Fo 86-70); primitive olivine
(Fo 91) is
only present in rare samples, even though all of the
picrites from a given locality with their own iron-rich
olivine phenocrysts are typically used in inferring
the presence of “olivine-controlled” liquids.
But it does not matter what the composition of olivine
happens to be – that is governed merely by some happenstance
of geology related to the detailed workings of an active
magma plumbing system. If the host groundmass or liquid
composition, or any mixing aliquot of it, was along
the cotectic, any backtrack calculation must involve
more than just olivine and cannot be uniquely determined.
Exhibiting
Olivine and Other Controls on CaO-Al2O3 Variation
Diagram
The simplest way to evaluate the geochemistry
is to use a diagram that is sensitive to addition/subtraction
of phases other than olivine. The usual FeO-MgO diagram
is not always by itself sufficient. A plot of CaO vs.
Al2O3 is effective (Figure 2).
The advantage of this diagram is that olivine plots
virtually at the origin (almost no Al2O3 or
CaO is present in olivine). Thus a nominal "olivine-controlled" set
of compositions, whether of glasses or whole-rocks,
should plot along a trend pointing toward the origin.
This is observed, for example in the olivine-controlled
glasses dredged from Kilauea-Puna Ridge (Clague
et al., 1995),
as it is on other variation diagrams.
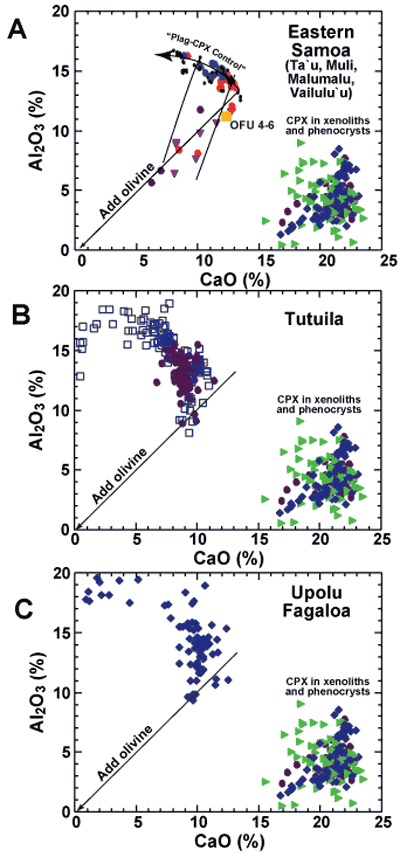
Figure 2: Al2O3 versus
CaO. Symbols are from Workman et al. (2004). A. Eastern
Samoa (Muli, Malumalu, and Vailulu`u Seamounts and
submerged and emergent Ta`u volcano. Red dots = whole-rock
analyses of samples with glass analyses; inverted
purple triangles = samples described as having clinopyroxene
in addition to olivine phenocrysts; blue dots = glasses;
large black dots = no petrographic information. Small
black dots = older analyses (GeoRoc data base). Orange
triangle = calculated Samoan parent from Putirka
(2008). Additional symbols are for clinopyroxene
in Type 2 magmatic xenoliths (green triangles) and
phenocrysts from Upolu (blue diamonds) and Tutuila
(black circles). Xenolith data are from Wright (1986,
1987), Dieu (1995) and J. Natland (unpublished).
A curving plagioclase-clinopyroxene differentiation
control curve is shown, the curving indicating different
proportions of the two minerals (more plagioclase)
as differentiation proceeds. An add olivine control
line extends toward the origin. The two diagonal lines
give possible olivine + clinopyroxene control trends
from either end of the array of glass compositions.
B. Filled circles = lavas of the Masefau basalt-dike
complex (Stearns, 1944); open squares = basalts of
the Greater Pago volcano of Natland (2003a; the Taputapu,
Pago, Pago-intracaldera, Alofau and Olomoana volcanoes
of Stearns, 1944). Xenoliths are as in A. C. Blue diamonds
= Fagaloa shield volcano; Xenoliths are as in A.
On the other hand, clinopyroxene,
the usual second silicate mineral to form in many seamount/island
basalts, plots at high CaO and moderately high Al2O3.
Trends of basalt or liquid compositions exhibiting
strong clinopyroxene control on the liquid line of
descent thus should plot along trends that move away
from the clinopyroxene field for phenocrysts as differentiation
involving clinopyroxene proceeds, or the trends should
lie between olivine at the origin (Figure 2, lower
left) and clinopyroxene (Figure 2, mid- to lower-right).
Figure 2A, in which I plot lava compositions from
eastern Samoa (Stice, 1969; Farley et
al., 1992; Workman et al., 2004), illustrates
the contrasting trends of differentiation well. These
data include compositions that fall along a nominal
olivine-control line pointing toward the origin (add-olivine,
in Figure 2A), and another trend among basalt liquids
almost orthogonal to this – the "Plag-CPX
Control" line, which applies to rocks with <7%
MgO contents. The latter are not "olivine controlled" but
their trend corresponds to the low- to intermediate-pressure
cotectic between plagioclase and clinopyroxene (shallow "gabbro" fractionation,
as first applied to Reykjanes Ridge near Iceland by Schilling,
1973).
Figure 2, A-C, show data from Eastern
Samoa (Ta`u Island, and Vailulu`u, Muli and Malumalu
Seamounts), Tutuila, and the Fagaloa volcano of Upolu,
respectively, which are progressively further west
along the Samoan chain. Only Eastern Samoa has samples
that plot along a potential "add-olivine" control
line. These are bulk-rock samples. Tutuila and Upolu,
which have another trend, can thus immediately be excluded
from further consideration. All existing analyses of
the rocks from these islands are multiply saturated
in at least olivine and clinopyroxene (Figure 1), and
thus any olivine-control-related assumptions do not
apply. The "picrites", so called, are what Macdonald (1949)
termed "picritic basalts of the ankaramite type" and
all of them contain clinopyroxene. That is, in thin
section they can be seen to contain abundant titanaugite
phenocrysts along with olivine (Figure 1). No
potential temperatures can be calculated from these
rocks by an olivine backtrack procedure. Some proportion
or aliquot of the rocks must have crystallized along
the low-pressure cotectic, and host melt compositions
would likely fall along a low-pressure cotectic, were
glass analyses available. Unfortunately, for Tutuila
and Upolu, there are no analyzed glasses. It
does not matter when, or in what mixed fraction of
a rock, clinopyroxene may have joined the liquidus.
Its mere presence is sufficient to show that this happened,
and that means there is no simple, let alone unique,
way to backtrack to an initial parental composition
using the bulk composition.
The minerals provide additional information
on this score. At Samoa, the olivine is differentiated
(Fo70-85);
it contains titanian magnesiochromite with substantial
iron as both FeO and Fe2O3; the
clinopyroxene is titanaugite; it contains crystals
of titanomagnetite. Individual samples usually contain
more than one discrete population of spinel and clinopyroxene.
These are unambiguously differentiated and hybrid rocks.
The only candidate for olivine control therefore
is eastern Samoa, where some of the rocks may have
only olivine phenocrysts. We shall test this. However,
note that little difference exists for analyses from
Eastern Samoa (Figure 2A) and basalts of either Tutuila
(Figure 2B) or Upolu (Figure 2C). And for eastern Samoa,
we need only evaluate those dozen or so samples that
fall along the “Add olivine” control line
in Figure 2A and are not so obviously, on this diagram,
clinopyroxene-bearing. They are broken down by symbols
as follows:
- those in which petrographic information in papers
listing the analysis states that there are both olivine
and clinopyroxene phenocrysts in the rock (inverted
purple triangles);
- those with olivine phenocrysts only (red circles),
but which have host cotectic (plagioclase-clinopyroxene)
glass compositions (blue circles); and
- those with only olivine phenocrysts but no corresponding
glass analyses (black circles; four samples)
First
note that all eastern Samoan glass samples (blue
circles) and most whole-rock samples (small black dots)
fall on or near the curving plagioclase-clinopyroxene
control arrow. This means that no olivine-controlled
Samoan glasses are known, even among bulk rocks, which
fall on or near to the “Add-olivine” line.
This leaves just four porphyritic
samples that cannot be evaluated using this diagram,
and these, to represent olivine-controlled liquids,
must lie significantly outside the domain of all known
Samoan glass compositions. However, all glasses that
are hosts for whole-rock compositions that plot along
the "Add olivine" line in Figure
2A have cotectic compositions (blue circles); that is,
bulk rocks with these known glass compositions are not
on “add olivine” lines. Instead, the bulk
rocks plot toward higher MgO because of addition of
clinopyroxene and olivine together along a general
trend bounded by the two diagonal lines in Figure 2A,
in typical proportions of about 4:1 or 3:1 olivine:clinopyroxene.
So, too, then, could the four similar black-dot samples
for which we have no glass compositions.
TiO2 versus CaO and Clinopyroxene Crystallization
To examine the influence of clinopyroxene
on these four remaining samples, CaO is plotted vs.
TiO2 for Eastern Samoan lavas and glasses
in Figure 3. In this diagram, olivine would plot at
the origin down and to the lower left, and off the
figure, and clinopyroxene off the figure to the upper
left. The olivine could have any Fo value, since CaO
and TiO2 are both
very low in any olivine. Any liquid may have olivine
added to it, as indicated by two "Add
olivine" lines, one at either end of the data
array for plagioclase-clinopyroxene cotectic liquids.
Truly primitive mixing liquids, or a liquid line of
descent from a very primitive starting composition,
would lie along or near the left "Add-olivine" line.
Olivine of any composition added to a more differentiated
liquid composition would follow some "Add-olivine" line
with a lower slope to the right. Bulk compositions
with olivine added to some intermediate cotectic liquid
composition would fall in between the two lines shown.
Most of the samples in question do. The light blue
field indicates these. Three of the four black-dot
samples are in this field. None of these are suitable
for backtrack calculations.
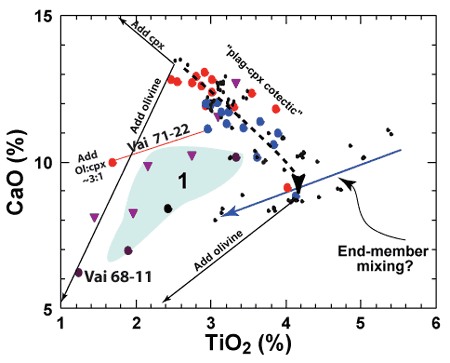
Figure 3: TiO2 versus
CaO contents of lavas from Eastern Samoa. Lava symbols
as in Figure 1A. Control and mixing lines are explained
in the text. One picritic sample, VAI 71-22 has both
a whole-rock and glass analysis; the red line connecting
the two indicates that it contains both olivine and
clinopyroxene phenocrysts in ~3:1 proportion. Field
1, light blue, bounds samples that either contain
both phenocrysts that mixed with cotectic glasses
(an array given approximately by the dashed arrow),
or which can have either olivine or olivine plus
clinopyroxene added to such differentiated compositions
(black dots).
An unusual trend exists at the high
TiO2 end of this diagram. Most glass samples
there form an array that points back obliquely to lower
TiO2 (blue arrow labeled “End-member
mixing”). These are liquids (glasses)
or aphyric whole-rock compositions that appear to be
hybrids combining something with very high TiO2 and
primitive picrite, with or without augite. This may
represent “end-member mixing”, and be related
to the physical isolation of magma bodies undergoing
extreme differentiation, and their later injection
with something straight out of the mantle, or nearly
so. Samoan magma chambers were not very big, and the
rate of eruption probably fairly low, to allow so much
differentiation to occur between injections of primitive
magma.
The black-dot samples that plot in the light blue
field are probably hybrids between intermediate cotectic
liquid and crystals of olivine, perhaps scavenged from
sludges somewhere beneath the volcanoes, as inferred
for picritic samples from Kilauea-Puna Ridge (Clague
et al., 1995). This reduces to one the number
of samples that could potentially be an olivine-controlled
liquid. This, at the lower left of the diagram, is
a primitive composition from the active seamount, Vailulu`u,
VAI 68-11. Since it is a primitive end-member in both
Figures 1A and 2, the identity of differentiated liquids
that may have mixed with it cannot be determined; it
could be the most primitive composition of all along
the left add-olivine line, or it could lie within an
extension of the light blue field and have an intermediate
cotectic glass composition.
Matthew Jackson (MIT/Woods Hole
Oceanographic Institution), who has studied these lavas,
sent me slides of three eastern Samoan picrites (personal
communication, 2008). Two of these turn out to have
some clinopyroxene phenocrysts. The other, VAI 63-11,
which has not been analyzed, contains fragments of
strained dunite, a type of deformed cumulate. All three
thin sections are thus of mixed or hybrid rocks, the
dunite being a type of xenolith. VAI 63-11 has enough
dunite and olivine phenocrysts so that it could plot
about where VAI 68-11 plots. Nevertheless, it is still
a mixed rock. Whether or not all the olivine in VAI
68-11 crystallized along a single liquid line of descent
is not yet established, but if it is, the sample would
uniquely not be
a mixed rock among Samoan picrites, and the only
one suitable for FeO-MgO olivine-liquid calculation
of a parental composition.
FeO-MgO Relationships Reconsidered
In Figure 4, MgO is plotted versus
FeO for the same three localities as in Figure 2, eastern
Samoa, Tutuila and Upolu. Here, FeO is taken to be
0.9 x FeOT (total iron as FeO; Herzberg & O’Hara,
2002), to allow for the likely oxidation state of the
rock. Regardless, at all three localities, basalts
have a range of FeO values at given MgO contents. The
samples with higher FeO are the ones used to weight
regressions and estimate or reconstruct “mantle” conditions
(e.g., Putirka,
2008). In Figure 3A, for Eastern Samoa,
the same symbols are used as previously. The range
of the “Plag-cpx" cotectic liquids (steep,
heavy arrow) spans the range of FeO contents of the “picrites”.
This high FeO is still taken by Putirka (2008) to
represent a liquid line of descent derived from a nominal
mantle with Fo91 olivine. For Samoa the
orange star (Samoa parent) shows this in Figure 4A.
Total iron as FeOT in this estimated parent
is 12%. This is an artificially high value, since everything
that went into its estimation is a hybrid rock.
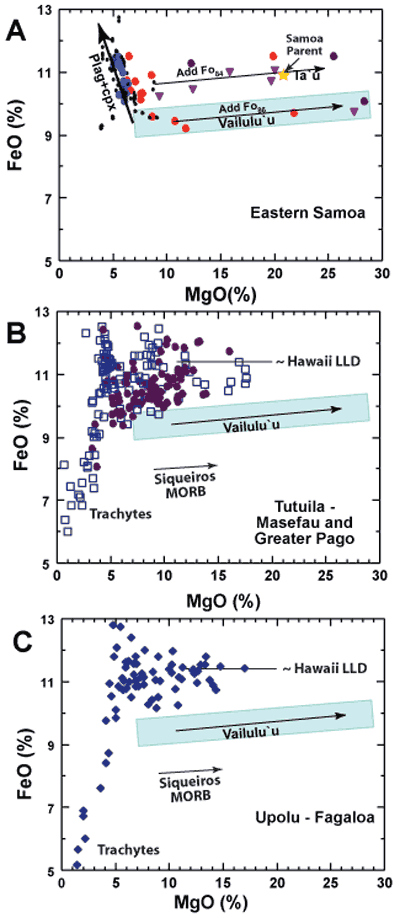
Figure 4: MgO versus FeO (= 0.9*
FeOT = total iron as FeO) for A. Eastern Samoa; B.
Tutuila; and C. Upolu. Symbols are as in Figure 1.
In A, samples from Vailulu`u seamount are highlighted
by the light blue field, which is repeated in B and
C. Fine arrows indicate trends of addition of olivine
phenocrysts with average compositions of Fo84 (upper
) and Fo86 (lower). Cotectic differentiation
of plagioclase and clinopyroxene is shown by the
bold arrow. The average Hawaiian calculated liquid
line of descent (Putirka, 2008) is shown in
B and C.
Alternatively, I argue that the “picrites” are
differentiated liquids to which olivine and augite
have been added by mixing. They have high FeO only
because the host liquids are not in equilibrium with
any of the olivine, and instead are differentiated
cotectic liquids. Alternatively, I argue that the “picrites” are
differentiated liquids to which olivine and augite
have been added by mixing. They have high FeO only
because the host liquids are not in equilibrium with
any of the olivine, and instead are differentiated
cotectic liquids (blue dots in Figure 4a). Eruptive
temperatures (Beattie, 1993) calculated for these glasses
are low, 1151ºC ± 21° (1118° – 1228°C;
n = 96). The values of “average olivine” that
need to be added are shown for two mixing arrows in
Figure 4A. These are not very primitive olivine compositions,
but are in accord with electron probe determinations
of the actual olivine in the picrites (Matthew
Jackson & Stan
Hart, personal communication, 2007), and also
with data on picritic basalts of the ankaramite type
from Tutuila and Upolu. They have compositions ranging
from Fo86-90. This range of olivine compositions
supports the idea that the parent for Vailulu`u seamount
(samples enclosed in the light blue field) is a hybrid
rock as just described, with Fo86-87 being
the likely composition of average “added olivine” to
a host liquid with about 7% MgO and 9.5% FeO (Figure
3A). This has total iron as FeOT of 10.55%,
nearly 1.5% less than the Samoan “parent” suggested
by Putirka (2008).
Why is Iron Low at Vailulu`u?
Why is Vailulu`u so much lower in
FeO? It may have to do with it being a small volcano
with, as yet, an insignificant amount of cotectic differentiated
material in the magma column. Ta`u, on the other hand,
is both bigger and older, and thus has a lot of cotectic
material in its magma column. Further west, Ofu Island,
which is now extinct, may have had even more differentiated
magmas its upper reaches when it was active (Natland,
2008). The system, as it develops, thus mulches on
itself, and develops extensive and extended high-iron
differentiation near its summit over time. Primitive
melts derived from the mantle necessarily interact
with this material in the magma column, and wind up
having enhanced total iron as FeOT as a
result of mixing. Such mixing has not occurred yet
at Vailulu`u.
Vailulu`u is thus a better indication
of temperature at Samoa than anywehere else, and it
must be at least 100-150°C lower than claimed by Putirka (2008) for Eastern Samoa. However, this is still guesswork because
none of these samples represent olivine-controlled liquids.
The Source
of ~Fo91
The most magnesian olivine found at
Vailulu`u is Fo90.2
(Matthew Jackson, personal communication,
2008). What is its source? Phenocrysts of >Fo88 are
rare among Samoan picrites. It is likely that differentiation
begins in the mantle and proceeds to shallow levels.
Thus, it is important to consider ultramafic xenoliths,
where such early differentiation may be recorded. This
has been done for xenolith suites from Tutuila, Upolu,
and Savai`i (Natland, 2008), of which there
are two types (Figure 5):
- (Type 1) residual xenoliths, representing
material, mainly harzburgite, from which basalt
was extracted at some point, and;
- (Type 2) high-P magmatic
segregations or cumulates, chiefly belonging to
a series comprising dunite, wehrlite, clinopyroxenite
and rare harzburgite. The clinopyroxene is green,
not pink or brown, so it is not titanaugite.
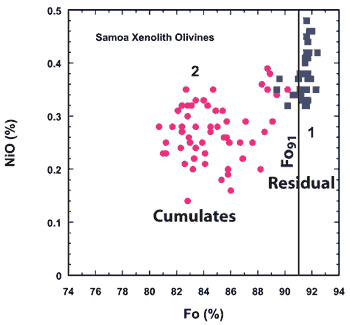
Figure 5: Olivine Fo versus NiO
content from Samoan ultramafic xenoliths. Type 1
= residual harzburgites, Type 2 = magmatic or cumulus
xenoliths. Data are from Wright (1986, 1987), Dieu
(1995) and J. Natland unpublished. The nominal mantle
value of olivine in equilibrium with the Samoan parent
of Putirka (2008) is Fo91, given by the vertical
line. This is more magnesian than any known magmatic
xenolith, and not as magnesian as the typical or
average olivine in residual harzburgite that underlies
most of the Samoan chain.
Although the olivine compositions
in Type 2 xenoliths extend back to most magnesian compositions
of about Fo90, even among such
xenoliths these are rare. In addition, almost all Type
2 xenoliths attest to the early and high-P cotectic
segregation of olivine and clinopyroxene together,
which violates the assumption of olivine control required
for the model of Putirka (2008) and studies
based on similar approaches. The overwhelming majority
of olivine crystals having Fo90-92 compositions,
however, are in Type 1 xenoliths.
Given the frequency with which xenocrysts
and xenoliths, particularly dunite, occur in Samoan
shield basalts, and without petrographic or mineralogic
proof to the contrary, the Fo90.2 in
the Vailulu`u sample could be a xenocryst scavenged
from either type of xenolith. Furthermore, given the
great frequency of mixing between low-P cotectic liquids
and other magmas with abundant accumulated olivine
and clinopyroxene, the likelihood is that
the Fo90.2 in the one sample from
Vailulu`u is not on
a unique line of descent linking it and its host liquid.
This could also be the case for any sample claimed
to represent a picritic mantle precursor related by
simple olivine control to all the other olivines in
the same sample, and the host liquid composition.
Conclusion: Conditions
for use of FeO-MgO olivine-liquid equilibria
The essential point is that mixing confounds this
subject. The evidence for mixing at Samoa (and, indeed,
at Hawaii and the Juan Fernandez Islands; Natland 2003b,
2007a,b) is so pervasive that it should be assumed
to have occurred unless explicitly disproven before
proceeding to model FeO-MgO olivine-liquid equilibrium.
Backtracking is viable only if the following general
conditions are met:
- The rocks are picrites, not ankaramites; they have
NO euhedral clinopyroxene or plagioclase phenocrysts;
- All olivine is faceted (euhedral) the picrites
do not contain olivine xenoclrysts or dunite indicative
of a mixing history;
- The olivine phenocrysts represent a single range
of compositions rather than clumping into two or
more discrete sets of composition;
- The same applies to spinel in olivine, which is
probably a better test;
- A bona fide Fo91 phenocryst is found,
not strongly zoned, euhedral, with an analyzed
inclusion of the unmodified (or at least easily
reconstructed) melt (glass) from which it precipitated;
- Host groundmass or glass compositions do not lie
along a plagioclase-clinopyroxene cotectic differentiation
trend;
- Proof (experimental or otherwise) that neither
clinopyroxene nor plagioclase were in equilibrium
with that crystal as well.
If these conditions are
met, the technique is likely to be valid and parental
MgO and Tp can be obtained. If any of these conditions
fail, they cannot.
Acknowledgements
I thank Matthew Jackson and Stan Hart
for providing thin sections and information on mineral
compositions from eastern Samoa; Don Anderson, and
Gillian Foulger for comments on an initial draft; Dean
Presnall, Trevor Falloon, and Yaoling Niu for reviewing
the manuscript; and Gill for shaping it up for the
web page.
References
-
Beattie, P., 1993. Olivine-melt
and orthopyroxene-melt equilibria: Cont.
Min. Pet.,
115, 103-111.
-
Breddam, K., 2002. Kistufell:
Primitive melt from the Iceland mantle plume: J. Petrol., 43,
345-373.
-
Clague, D.A., Moore, J.G., Dixon,
J.E., and Friesen, W.B., 1995. Petrology of submarine
lavas from Kilauea’s Puna Ridge, Hawaii. J. Petrol., 36,
299-349.
-
Clague, D.A., Weber, W.S. and Dixon, J.E.,
1991. Picritic glasses from Hawaii. Nature, 353,
553-556.
-
Daly, R.A., 1924. The geology of American
Samoa. Carnegie
Institution of Washington, Publication 340:
5-145.
-
Dieu, J., 1995. On the formation of cumulates,
characteristics of oceanic lithosphere, and the
process of carbonatite metasomatism; a study
of ultramafic xenoliths from South Pacific Islands.
University of California, San Diego (Ph.D. Dissertation),
393 pp.
-
Drever, H.I., & Johnston, R., 1958. The
petrology of picritic rocks in minor intrusions – a
Hebridean group. Trans. Royal Soc. Edinburgh, 63,
459-499.
-
Falloon, T.J., Danyushevsky, L.V., Ariskin,
A., Green, D.H., and Ford, C.E., 2007a, The application
of olivine geothermometry to infer crystallization
temperatures of parental liquids: implications
for the temperatures of MORB magmas, Chem.
Geol., 241, 207-233.
-
Falloon,
T.H., Green, D.H. and Danyushevsky, L.V., 2007b.
Crystallization temperatures of tholeiitic parental
liquids: Implications for the existence of thermally
driven mantle plumes. In Foulger, G.R., and Jurdy,
D.M. (eds.), Plates,
Plumes, and Planetary Processes. Geological
Society of America Special Paper 430, 235-2652.
-
Falloon,
T.H., Green, D.H., Danyushevsky, L.V., and McNeill,
A.W., 2008. The composition
of near-solidus partial melts of fertile peridotite
at 1 and 1.5 GPa: Implications for the petrogenesis
of MORB. J. Petrol., 49: 591-613,
doi:10.1093/petrology/egn009.
-
Farley, K.A., Natland,
J.H., and Craig, H., 1992. Binary mixing of enriched
and undegassed (primitive?) mantle components (He,
Sr, Nd, Pb) in Samoan lavas. Earth Planet. Sci.
Lett., 111,
183-199, doi:10.1016/0012-821X(92)90178-X.
-
Francis, D., 1985.The Baffin
Bay lavas and the value of picrites as analogues
of primary magmas. Cont. Min. Pet.,
89: 144-154.
-
Gurenko,
A.A., and Chaussidon, M., 1995. Enriched and depleted
primitive melts included in olivine from Icelandic
tholeiites: Origin by continuous melting of a single
mantle column, Geochim.
et Cosmochim. Acta, 59,
2905-2907, doi:10.1016/0016-7073(95)00184-0.
-
Hansteen,
T.H., 1991. Multi-stage evolution of the picritic
Maelifell rocks, SW Iceland: constraints from mineralogy
and inclusions of glass and fluid in olivine: Cont.
Min. Pet., 109,
225-239.
-
Hart, S.R., Coetzee, M., Workman, R.K., Blusztajn,
J., Johnson, K.T.M., Sinton, J.M., Steniberger,
B., and Hawkins, J.W., 2004. Earth Planet.
Sci. Lett., 227, 37-56.
-
Helz,
R.T., 1987. Diverse olivine types in lava of the
1959 eruption of Kilauea volcano and their bearing
on eruption dynamics. In: Decker, R.W., Wright,
T.L., & Stauffer, P.H. (eds), Volcanism
in Hawaii, U.S. Geological Survey Professional
Paper 1350. Washington: U.S.
Government Printing Office, 691-722.
-
Herzberg, C.,
Asimow, P.D., Arndt, N., Niu, Y., Lesher, C.M.,
Fitton, J.G., Cheadle, M.J., and Saunders, A.D.,
2007. Temperatures in ambient mantle and plumes:
Constraints from basalts, picrites, and komatiites. Geochemistry,
Geophysics, Geosystems, 8,
Q02006, doi:10.1029/2006GC001390.
-
Herzberg, C. and O’Hara,
M.J., 2002, Plume-associated magmas of Phanerozoic
age, J. Petrol., 43,
1857-1883.
-
Jackson, M.G., Hart, S.R., Saal, A.E.,
Shimizu, N ., Kurz, M.D., Blustajn, J.S. and Skovgaard,
A.C., 2008. Globally elevated titanium, tantalum
and niobium (TITAN) in ocean island basalts with
high 3He/4He, Geochemistry, Geophysics, Geosystems,
9: doi:10.1029./2007GC001876, 1-21.
-
Kear, D., and
Wood, B.L., 1959. The geology and hydrology of Western
Samoa. N. Zeal. Geol.
Surv. Bull., 63, 92 p.
-
Kirkpatrick, R.J., 1979. Processes
of crystallization in pillow basalts, Hole 396B,
DSDP Leg 46. In: Dmitriev, L., Heirtzler,
J., et al., Initial Reports of the Deep Sea
Drilling Project 46. Washington: U.S. Government.
Printing Office, 272-282.
-
Komar, P.D., 1972. Mechanical interactions
of phenocrysts and flow differentiation of igneous
dikes and sills. Geol. Soc. Am. Bull., 83,
973-988.
-
Larsen, L.M., and Pedersen, A.K, 2000.
Processes in high-Mg, high-T magmas: Evidence from
olivine, chromite and glass in Paleogene picrites
from West Greenland, J. Petrol., 41,
1071-1098.
-
Macdonald, G.A., 1949. Hawaiian petrographic
province, Bull. Geol. Soc. Am., 6,
1541-1596.
-
Natland, J.H., 1980a. Progression
of volcanism in the Samoan linear volcanic chain.
In: Irving,
A.J. and Dungan, M. (eds.), The Jackson Volume, Amer.
J. Sci., 280-A, 709-735.
-
Natland,
J.H., 1980b. Crystal morphologies
in basalts dredged and drilled from the East
Pacific Rise near 9°N and the Siqueiros Fracture
Zone. In Rosendahl, B.R., Hékinian,
R., et al., Initial Reports of the Deep Sea Drilling
Project: Washington (U.S. Government Printing
Office), 605-633.
-
Natland, J.H., 1989. Partial
melting of a lithologically heterogeneous mantle:
Inferences from crystallization histories of magnesian
abyssal tholeiites from the Siqueiros Fracture
Zone. In: Saunders, A.D. and Norry, M. (eds.), Magmatism
in the Ocean Basins, Geol. Soc. London, Spec.
Publ., 42, 41-77
-
-
Natland,
J.H., 2003b. Capture of mantle helium by growing
olivine phenocrysts in picritic basalts from the
Juan Fernandez Islands, SE Pacific. J.
Petrol. 44, 421-456.
-
Natland,
J.H., 2007a. 12 January 2007 Discussion of: Crystallization
temperatures of tholeiite parental liquids: Implications
for the existence of thermally driven mantle plumes
by Trevor Falloon, D.H. Green & L.V. Danyushevsky.
In Foulger, G.R., and Jurdy, D.M., (Eds.), Plates,
Plumes and Planetary Processes, Geological
Society of America Special Paper 430. 252-253.
-
Natland,
J.H., 2007b. 31 January 2007 Discussion of: Crystallization
temperatures of tholeiite parental liquids: Implications
for the existence of thermally driven mantle plumes
by Trevor Falloon, D.H. Green & L.V. Danyushevsky
. In Foulger, G.R., and Jurdy, D.M., (Eds.), Plates,
Plumes and Planetary Processes, Geological Society
of America Special Paper 430, 255-256.
-
Natland,
J.H., 2008. Will the real Fo91 please
stand up?, in preparation.
-
O’Hara, M.J., 1968. The
bearing of phase equilibria studies on the origin
and evolution of igneous rocks. Earth-Science Reviews, 4,
6-133.
-
Perfit, M.R., Fornari, D.J., Ridley, W.I.,
Kirk, P.D., Casey, J., Kastens, K.A., Reynolds, J.R.,
Edwards, M., Desonie, D., Shuster, R., and Paradis,
S., 1996. Recent volcanism in the Siqueiros ransform
fault: picritic basalts and implications for
MORB genesis. Earth Planet. Sci. Lett., 141,
91-108.
-
Presnall, D. C., and Gudfinnsson,
G.H., 2008. Origin of oceanic lithosphere. J.
Petrol., 49,
615-632, doi:10.1093/petrology/egm052
-
Putirka, K.,
Perfit, M., Ryerson, F.J., and Jackson, M.G., 2007.
Ambient and excess mantle temperatures, olivine thermometry
and active vs. passive upwelling, Chem.
Geol., 241, 177-206, doi:
10.1016/j.chemgeo.2007.01.014.
-
-
Robillard, I., Francis,
D., and Ludden, J.N., 1992. The relationship
between E= and N-type magmas in the Baffin Bay
lavas. Cont. Min. Pet., 112:
230-241.
-
Roeder, P.L., and Emslie, R.F.,
1970. Olivine-liquid equilibria. Cont. Min.
Pet., 29,
275-289.
-
Schilling, J.-G., 1973a, Iceland
mantle plume - geochemical study of Reykjanes ridge, Nature, 242,
565-571.
-
Simkin, 1967.
Flow differentiation in the picritic sills of north
Skye, In Wyllie, P.J.
(Ed.), Ultramafic and Related Rocks,
New York (John Wiley & Sons), 64-69.
-
Stearns,
H.T., 1944. Geology of the Samoan Islands. Geol.
Soc. Am. Bull., 55, 1279-1332
-
Stice, G.D.,
1968. Petrography of the Manu`a Islands, Samoa, Cont.
Min. Pet., 19,
343-357.
-
Workman, R.K., Hart, S.R., Jackson, M.G.,
Regelous, M., Farley, K.A., Blustajn, J, Kurz,
M., and Staudigel, H., 2004. Recycled metasomatized
lithosphere as the origin of the Enriched Mantle
II (EM2) end-member: Evidence from the Samoan volcanic
chain, Geochemistry, Geophysics, Geosystems, 5,
Q04008, doi:10.1029/2003GC000623.
-
Wright, E., 1986. Petrology
and geochemistry of shield-building and post-erosional
lava series of Samoa; implications for mantle
heterogeneity and magma genesis. University
of California, San Diego (Ph.D. Dissertation),
305 pp.
-
Wright, E., 1987. Mineralogical studies of
Samoan ultramafic xenoliths: implications for upper
mantle processes. In Fryer, P., Batiza, R., and
Boehlert, G.W. (eds.), Seamounts, Islands,
and Atolls, Geophysical Monograph, 43: Washington
(American Geophysical Union), 221-234.
last updated 1st
September, 2008 |