 |
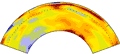
Hawaii cross section
|
Plumes
or reheated slabs?
|
Statement of the problem
Understanding
convection in the Earth’s mantle is a primary
prerequisite for understanding planetary evolution.
It has long been debated whether the whole mantle
convects whether separate lower- and upper-mantle
convection occurs, or both [1, 2].
In 1971 Morgan proposed the so-called, plume mode
of convection [3]. Plumes,
hypothetical hot buoyant structures, were postulated
to represent uprising convective flow penetrating
the whole mantle. Originally they were considered
to rise subvertically from the lower mantle, break
through the rigid lithospheric plates, and move subhorizontally
providing the driving force for plate tectonics. Numerous
computer simulations utilising dimensionless numbers,
and analogue laboratory experiments on materials such
as paraffin, have shown the primary characteristics
of plume mode convection – a large head and
a thin conduit (see [1] for a general review, and
Figure 1a for a typical representation of a plume
[4]).
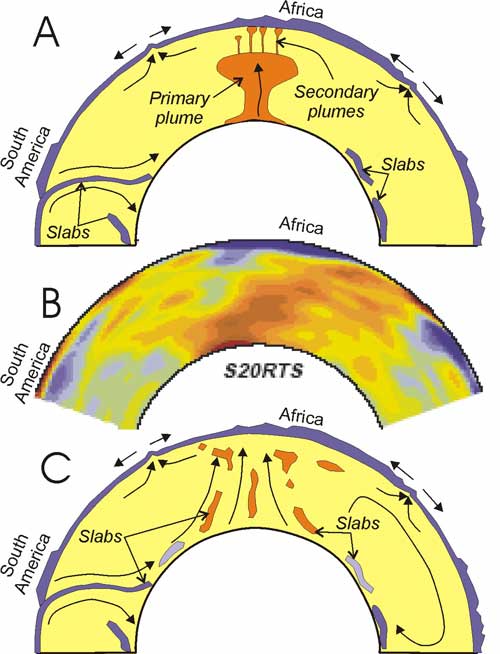
Figure. 1. Comparison
of (a) mantle plume convection, (b) the observed structure
of the mantle (from Ritsema et al., 1999) and (c)
penetrative convection. Drawings are slightly modified
from [4],
[8]
and [14] respectively. Bluish, yellow and brownish
colors represent high-, normal- and low-velocity anomalies,
respectively.
The clear postulates
of the convection plume model, and logical explanation
in the framwork of plate tectonics for the unidirectional
migration of volcanism in some oceanic chains (e.g.
Hawaii) and continental
volcanic fields (e.g. Yellowstone)
persuaded the majority of researchers, the author
being no exception, to believe that plumes do exist.
Consequently, the number of volcanic fields explained
by plumes as the primary source of magma grew from
about 20 [3] to up to
5,200 in the extreme [5].
However, as the number
of “plume-related” volcanic fields increased,
the controversies and circular reasoning involved
became obvious [6, 7].
One example is deep seismic tomography models, such
as those presented by Ritsema and co-authors [8,
9]
(Figure 1b). The large low-velocity anomalies in these
models do not resemble envisaged plume structures,
but are more similar to the high-velocity anomalies
which are interpreted as subducted slabs by almost
all researchers. Care must be taken, however, because
the resolution of deep seismic tomography models is
currently low [9]
and there is a tendency for tomographic methods to
widen artificially vertical anomalies in the lower
mantle [10].
What if the shapes of
large low-velocity anomalies in the mantle are real?
Certainly they cannot then be explained in terms of
classical plume model, because 1) they are too deflected
from the vertical compared with what is expected [11]
and 2) they are not circular in cross section, but
instead they are sheet-like. Having noted these features,
S.O. Balyshev and I postulated that such anomalies
might be ancient heated lithospheric plates (slabs)
buried in the mantle as a result of subduction [12].
The concept that a velocity inversion could develop
in a subducted slab as it warms up and partially melts,
encouraged by the presence of volatiles, was originally
suggested by T. Gasparik in 1997, who viewed this
process as occurring in the upper mantle [13].
To change dense, cold slabs to low-density, and thus
low-velocity structures, we explored radioactivity
as a source of heating.
Radioactivity calculations
We used the following
initial assumptions for the calculations:
-
The radioactive
elements K, Th and U are distributed within a 1-km-thick
sedimentary layer in the oceanic crust;
-
The abundances of
radioactive elements in oceanic sediments in the
past were the same as in present-day pelagic sediments;
-
Loss of radioactive
elements during subduction through partial melting
and degassing of subducted sediments did not exceed
50%; and
-
The time required
to account for the observed enriched Sr-Nd-Pb-isotopic
features of most intraplate oceanic basalts is of
the order of 1-2 billion years.
With these assumptions,
heating of the subducted slab is easily possible [12].
In Figure 1c the penetrative
convection model is shown, taken from the original
publication of Silver et al. [14]. In this
model cooling and subduction of oceanic plates is
the driving force for mantle convection. In reality,
the subducting slabs are themselves the downwelling
convective flows. Silver et al. [14] assumed
that buried mantle slabs warm to reach the ambient
temperature of the mantle and at that time become
buoyant because of the difference in chemical composition
between the slab and the mantle. When buoyant, slabs
start to rise from the lower mantle towards upper
mantle. In this way, the reheated slabs comprise the
upwelling convective flows. To my knowledge, the conversion
of dense slabs to low-density structures at ambient
mantle temperatures had not been tested. However,
radiogenic heating of the slabs strengthens the model
of penetrative convection. If it is correct, plate
tectonics acquires a primary role in convection on
the scale of the whole mantle.
When re-examining seismic
tomography images of the mantle (Figure 1b) in the
light of the penetrative convection concept, a candidate
explanation for at least two of the most prominent
low-velocity structures becomes immediately obvious
– the anomalies are sheet-like because they
are ancient, reheated subducted slabs.
Detailed discussion
of the radiogenic isotope geochemistry of oceanic
basalts that is commonly used to support the plume
hypothesis is beyond the scope of this brief article.
Nevertheless, a few comments are worthwhile. In our
paper [12]
S.O. Balyshev and I repeated the common mistake of
geochemists that high 3He/4He
ratios can be used as reliable tracer of a lower mantle
contribution. Indeed, this is not the case [15,
16]. Other isotopes,
for instance Sr-Nd-Pb isotopes, can say nothing about
a lower mantle contribution either. According to conventional
geochemical interpretations, three of the four so-called
mantle tetrahedron end-members EM1, EM2 and HIMU,
are considered to be related to the subduction of
continental lithosphere, pelagic sediments and oceanic
lithosphere, respectively ([17] and references therein).
In many standard plume models these components are
thought to be stored at either the core-mantle- or
lower-upper-mantle boundaries for prolonged intervals
of time (1-2 billion years) where they attain their
highly evolved radiogenic-to-stable isotope ratios
(e.g. low 143Nd/144Nd for EM1,
high 87Sr/86Sr for EM2 and high
207Pb/204Pb and 206Pb/204Pb
for HIMU). They are then sampled at these depths by
mantle plumes. Again, if we re-examine the data from
the point of view of penetrative convection, there
is no need for plumes at all to transport these components
to the surface, and we do not need to change the geochemical
interpretations. Heated, uprising slabs can deliver
these components to the surface instead of plumes.
Discussion
Gasparik [13]
attributes the conversion of seismic anomalies in subducted
slabs to the development of small amounts of melt within
them. Anderson [18]
suggests that melts can develop within slabs as a consequence
of conductive heating of the slab by ambient mantle
and that the time scale for this process is of the order
of 20 Myr or less. Indeed this is plausible explanation.
However, some older, slab-like low-velocity anomalies
are
clearly visible in the mantle. For example van der
Voo et al. [19] suggest that a high-velocity anomaly
beneath Siberia is a Mesozoic slab.
Experimental data suggest
that water-bearing minerals (e.g., superhydrous
phase B) may survive within a slab up to the depths
below upper-lower mantle transition zone and hence can
provide significant flux of water into the lower mantle.
Under greater depths these minerals provide water for
Mg- and Ca-perovskite and magnesiowustite, the major
water concentrators in the lower mantle ([20] and references
therein). These minerals will dehydrate at different
P-T conditions. Thus, slabs probably convert to low-
and high-velocity anomalies several times during their
storage in the mantle while degassing and melting because
of conductive and further radiogenic heating.
When discussing the two
largest low-velocity anomalies in the present day mantle,
the African and South Pacific anomalies, we should also
keep in mind that ancient ages are expected for them
from enriched isotopic features of “hot spot”
volcanic rocks. These anomalies cannot be related to
recent subduction zones and hence if they result from
velocity conversions, a radiogenic source of heating
is still required.
Summary
The concept of penetrative
convection in my view is logical and requires fewer
ad hoc assumptions than the mantle plume
model does at present. However, the model is not yet
fully developed or understood. We nevertheless re-introduce
it to highlight that it has to be thoroughly considered
from different points of view, including physical
modeling, 3D seismological probing and geological
testing.
|
[1] Davies G.F., Richards
M.A. Mantle convection. J. Geology, 100,
151-206, 1992.
[2] Anderson D. Mantle convection – Convection.html
[3] Morgan W.J. Convection
plumes in the lower mantle. Nature, 230,
42-43, 1971.
[4] Courtillot
V., Davaille A., Besse J., Stock J. Three distinct types
of hotspots in the Earth’s mantle. Earth. Planet.
Sci. Lett., 205, 295-308, 2003.
[5] Malamud B.D., Turcotte D.L. How many plumes are
there? Earth Planet. Sci. Lett., 174,
113-124, 1999.
[6] Sheth H.C. Flood basalts and large igneous provinces
from deep mantle plumes: fact, fiction, and fallacy.
Tectonophysics, 311, 1-29,
2001, and Deccan.html
[7] Anderson D.L. The plume assumption: frequently used
arguments – FUA.html
[8] Ritsema
J., van Heijest H.J., Woodhouse J.H. Complex shear velocity
structure imaged beneath Africa and Iceland. Science,
286, 1925-1928, 1999.
[9] Ritsema
J., Allen R.M. The elusive mantle plume. Earth Planet.
Sci. Lett., 207, 1-12, 2003.
[10] Dziewonski
A.M. Global seismic tomography: what we really can say
and what we make up. Abstract in The Hotspot Handbook,
Proceedings of Penrose Conference Plume IV: Beyond the
Plume Hypothesis, Hveragerdi, Iceland, August 2003.
[11] Richards M.A., Griffiths R.W. Deflection of plumes
by mantle shear flow: experimental results and a simple
theory. Nature, 342, 900-902,
1988.
[12] Balyshev S.O.,
Ivanov A.V. Low-density anomalies in the mantle: ascending
plumes and/or heated fossil lithospheric plates? Doklady
Earth Sciences, 380, 858-862,
2001.
[13] Gasparik,
T., A model for the layered upper mantle, Phys.
Earth Planet. Int., 100, 197-212,
1997.
[14] Silver P.G., Carlson R.W., Olson P. Deep slabs,
geochemical heterogeneity, and the large-scale structure
of mantle convection: Investigation of an enduring paradox.
Ann. Rev. Earth. Sci., 16,
477-541, 1988.
[15] Meibom A.,
Anderson D.L., Sleep N.H., Frei R., Chamberlain C.P.,
Hren M.T., Wooden J.L. Are high 3He/4He
ratios in oceanic basalts an indicator of deep-mantle
plume components? Earth Planet. Sci. Lett.,
208, 197-204, 2003.
[16] Anderson D.L., Foulger G.R., Meibom A. Helium:
Fundamental models – HeliumFundamentals.html
[17] Dickin A.P. Radiogenic isotope geology.
Cambridge University Press, 490 p., 1997.
[18] Anderson D.L. Reheating slabs by thermal conduction
in the upper mantle, HotSlabs2.html
2003.
[19] van der Voo R., Spakman W., Bijwaard H. Mesozoic
subducted slabs under Siberia, Nature, 397,
246-249, 1999.
[20] Litasov K., Ohtani E. Stability of various hydrous
phases in CMAS pyrolite-H2O system up to
25 GPa, Phys. Chem. Minerals, 30,
147-156, 2003.
last updated 17th November,
2005 |
|
|